Polyethylene, High Density (HDPE)
INTRODUCTION
For normal molecular weight polyethylenes (melt index ] 0.5), the density of homopolymer HDPE is fixed at 59.9-60.2 lb/ft3 (0.960 to 0.965 g/cm3), depending on the manufacturing process. However, HDPE spans the density range of 58.7-60.3 lb/ft3 (0.941 to 0.967 g/cm3) by the use of copolymers that add side-chain branches and thus reduce the density.
The density of HDPE is controlled in the manufacturing process by the amount of comonomer added to the reactor. Typical comonomers used with ethylene in HDPE are propylene, butene, hexene, and octene. As the molecular weight of polyethylene increases, the longer polymer chains do not crystallize as readily and the lower amount of crystallinity further reduces the density of a HDPE homopolymer (melt index [ 0.5).
CATEGORY
HDPE is a partially crystalline, partially amorphous thermoplastic material. The degree of crystallinity depends on the molecular weight, the amount of comonomer present, and the heat treatment given. The crystallinity of a given HDPE resin can be varied over a wide range by the rate of cooling from the molten state; slower cooling rates favor crystalline growth. The range of crystallinity for HDPE is normally 50-80%. A density value normally quoted on data sheets for HDPE is determined by a compression molded sheet that has been cooled at the rate of 27°F (15°C) per minuted. Most commercial fabrication processes cool from the melt at much faster rates; as a result, an article fabricated from HDPE rarely reaches the density quoted on a data sheet. Because the amount of crystallinity in HDPE is variable, HDPE can be considered as an amorphous polymer having a variable amount of crysstalline filler.
HISTORY
The introductory section on polyethylenes provides historical information on HDPE.
POLYMERIZATION
HDPE is manufactured by a low pressure process; by comparison, low density polyethylene (LDPE) is manufactured by a high pressure process. The pressure used in manufacturing HDPE is below 2000 psi (14 MPa); in many cases, it is below 1000 psi (7 MPa). [In manufacturing LDPE, pressures commonly exceed 10,000 psi (70 MPa)].
There are three major commercial processes used for polymerization of HDPE: solution, slurry, and gas-phase processes. The catalysts used in the manufacture of HDPE are usually either a transition metal oxide type or a Ziegler/Natta type. It is important to note that the performance of HDPE resins having identical melt indexes, densities, and molecular weight distributions (MWDs) can vary if the resins are produced by different processes. These differences normally are seen only in critical applications having very narrow processing windows. For most applications, HDPE resins selected from more than one supplier will perform adequately, even if the resins are made by different processes.
As mentioned, along with melt index and density, MWD is a distinguishing property of HDPE. As HDPE is polymerized, polymer molecules of many different lengths (molecular weights) are produced. If an HDPE resin has a narrow range of molecule lengths, it is said to have a narrow MWD. Conversely, an HDPE having a broad range of molecule lengths is said to have a broad MWD. The MWD is a plot of molecular weight versus the number or frequency of a given molecular weight. As the MWD of an HDPE is broadened, the processibility and melt strength increase, while impact strength, low-temperature toughness, and warpage resistance decrease. The MWD of HDPE is largely controlled by the type of catalyst used in polymerization and by the type of manufacturing process employed.
DESCRIPTION OF PROPERTIES
In HDPE, the properties of tensile yield strength, stiffness, creep resistance, impermeability, abrasion resistance, mold shrinkage, and hardness increase with increasing density. On the other hand impact strength, flexibility, and environmental stress crack resistance (ESCR) increase with decreasing density.
As the average molecular weight of HDPE increases, the polymer's molten flow decreases. The standard test for measuring the molten flow of HDPE is called the melt index. Melt index is inversely proprotional to the average molecular weight. The properties of ESCR, impact strength, tensile strength, elongation, melt strength, and die swell improve with decreasing melt index (and with increasing average molecular weight). The properties of processibility, melt drawdown, and optics decrease with decreasing melt index. As the average molecular weight of HDPE increases, there is more shrinkage and warpage present in the molded parts.
These brief comments on melt index and density indicate that it is not possible to maximize all of the properties of HDPE in a single resin. Therefore, compromises are necessary in designing any HDPE resin. For that reason, most manufacturers of HDPE offer many different HDPE resin grades in their product mix. Selection of an HDPE resin for a given application involves careful evaluation of the application requirements, in order to select the HDPE resin that most closely satisfies the most important requirements.
The glass-transition temperature Tg of polyethylene is well below room temperature. This gives polyethylene its more rubbery nature (compared to a polymer such as polystyrene which has a glass-transition point above room temperature). The rubbery nature of HDPE also limits its service temperature compared to that of a polymer having a Tg above room temperature. The Tg for HDPE has been assigned several different values by different measuring techniques and is the subject of much controversey. There are three temperature ranges commonly assigned as the glass-transition point for polyethylene: -207 to - 171°F (-133 to -113°C), -126 to -99°F (-88 to -73°C), and -45 to 9°F (-43 to -13°C).(5)
Because HDPE is rubbery, its creep modulus is more important than, for example, its flexural modulus in determining the in-service strength of a part fabricated from it. In designing an HDPE part that is intended to bear a load for an extended period of time (greater than one hour), flexural or tensile modulus cannot safely be used to calculate the strength of the fabricated part. Instead, the designer should refer to creep data and select a creep modulus that corresponds to the maximum service time under load for the part. The creep modulus should be employed in strength calculations instead of the flexural and tensile modulus.
Failure to consider creep when parts are designed in HDPE is an invitation to premature part failure. Creep resistance improves in HDPE with increasing density and increasing average molecular weight. Creep resistance can also be improved by the use of such cross-linking techniques as irradiation and chemical cross-linking.
APPLICATIONS
HDPE is used for many food packaging applications because it provides excellent moisture barrier properties. However, HDPE, like all polyethylenes, is limited to those food packaging applications that do not require an oxygen barrier. In film form, HDPE is used in snack food packages and cereal box liners; in blow-molded bottle form, for milk and some non-carbonated beverage bottles; and in injection-molded tub form, for packaging margarine, whipped toppings, and deli foods.
Because HDPE has good chemical resistance, it is used for packaging many household as well as industrial chemicals. Examples of such injection-molded applications include 5-gal pails of floor cleaner, 1-gal pails of paint, and construction containers of spackling paste. Blow-molded applications include 55-gal HDPE drums of antifreeze.
Although HDPE has good chemical resistance, it is prone to environmental stress cracking (ESC), commonly from such agents as detergents and surfactants. HDPE can still be used to package these items if the designer carefully selects an HDPE with a time-to-ESC-failure longer than the required shelf life of the product. Resistance to ESC for HDPE increases with decreasing density and melt index. Although there are many tests for ESC resistance, there is no substitute for field experience with a given product.
HDPE does not provide good barrier resistance to lower molecular weight hydrocarbon solvents such as kerosene, and it is not recommended for nonvented packages of such solvents. HDPE is used for some noncritical gasoline containers where the loss of gasoline through the wall can be tolerated and the container is vented. It can be used for critical gasoline containers, such as automotive gas tanks, if a barrier is added-by surface sulfonation and fluorination treatments, for example.
General uses of HDPE include injection-molded beverage cases, bread trays, and dunnage trays as well as films (which have wide and growing use in merchandising and grocery sacks). Another interesting HDPE application is extruded sheet that is subsequently thermoformed into such articles as canoes and pickup truck bed liners. HDPE can readily be thermoformed but not as easily as styrenics.
In sum, HDPE is a versatile thermoplastic enjoying many successful applications that maximize its properties.
ADVANTAGES/DISADVANTAGES
The performance attributes of HDPE have earned this resin family significant commercial uses. Compared to LDPE and LLDPE, HDPE provides greater stiffness and rigidity.
Advantages
- Good moisture barrier properties beneficial in many packaging applications (inner-liner films in paperboard cartons, molded end caps for caulking tubes)
- Good stiffness adequate for some structural applications (beverage crates, tote bins, pallets)
- Load-bearing applications when creep is factored correctly in the part design (load-bearing capabilities improve with increasing molecular weight; ultra-high molecular weight HDPE affords the greatest load-bearing capabilities)
- Relative chemical inertness (can be used to package some chemicals)
- Good thermal stability over a range of - 40 to 600°F (-40 to 316°C).
Disadvantages
- Relatively high gas transmission rates (would not protect a packaged product from oxygen penetration)
- The creep propensity of HDPE prevents it from being considered a true engineering plastic; the load-bearing capability of conventional molecular weight HDPE decreases rapidly with increasing environmental temperature
- Some chemicals may cause premature failure of HDPE parts because of ESC. Prior to packaging chemicals in containers fabricated of HDPE, testing is recommended as some solvents will penetrate and soften HDPE
- Higher temperatures may cause degradation of HDPE unless an antioxidant is added to the resin
PROCESSING TECHNIQUES
HDPE can be made into film using both the extrusion blown film and cast film processes. It is efficiently extruded into sheet and profiles and is readily injection molded, blow molded, and rotationally molded. When a blowing agent is added, HDPE can be fabricated into foamed products. HDPE can also be compression molded, although it is rare to find a commercial system doing so. HDPE can be forged and molded at temperatures below its melting point, but this is not done commercially. Promising developmental work is under way on the production of fibers from HDPE.
As HDPE is fabricated into film and other articles, orientation-influenced properties become very significant. The crystalline structure of HDPE is oriented during the fabrication process; as a result, films or articles exhibit characteristically anisotropic physical properties.
For example, the impact properties a test specimen cut from an injection-molded part parallel to the line of major flow of plastic into the mold would be significantly different from those of a test specimen cut perpendicular to the major line of flow into the mold. This anisotropic behavior must be considered in HDPE part design. If the fabrication process cannot minimize the anisotropic behavior of fabricated HDPE, then the film or article should be designed to take advantage of the orientation. Fabrication methods commonly used with HDPE are listed in the Master Material Outline.
Most fillers and reinforcing agents can be used with HDPE. The addition of a filler usually improves flexural strength, creep resistance, and hardness, but such gains in physical properties may be offset by reduced impact strength, tensile elongation, and processibility. The addition of a filler can also increase the density of HDPE, and increased density can be a detriment rather than a gain.
Most HDPE resins are sold by the pound by resin producers to fabricators, who, in turn, sell the fabricated articles by the part, not by the pound. The resin producer is concerned with weight, while the seller of fabricated parts is concerned with weight and volume. This relationship of weight and volume can become critical when the density of HDPE (volume per pound) is increased by adding a filler. Any increase in density means that a fabricator must buy (or process) more pounds of filled HDPE to make the same number of parts. This can result in a hidden cost to the fabricator for filled HDPE.
Compounding is another cost factor in filled HDPE systems. Most fillers cannot simply be blended with HDPE and used on normal fabrication equipment. The HDPE and the filler must be compounded by extrusion, intensive mixers, or both, before fabrication, which can add 6 to 12 cents per pound to the cost of filled HDPE. Because of the relatively low cost of unfilled HDPE, and the hidden costs of increased density and compounding, it is difficult to add a filler to HDPE and improve economics. This would not be so, of course, if the cost of HDPE exceeded $1 per pound or if the natural density of HDPE were greater than 62 lb/ft3 (1 g/cm3). Some fillers used with HDPE are talc, calcium carbonate, and mica.
Processability of HDPE
|
Process |
A |
B |
C |
Injection Molding |
X |
|
|
Extrusion |
X |
|
|
Thermoforming |
X |
|
|
Foam Molding |
X |
DIP. Slush Molding |
|
|
|
Rotational Molding |
X |
|
|
Powder Fluidized-Bed Coating |
|
|
|
Reinforced Thermoset Molding |
|
|
|
Compression/Transfer Molding |
|
|
X |
Reaction Injection Molding (RIM) |
|
|
|
Mechanical Forming Casting |
|
|
X |
A = Common processing technique.
B = Technique possible with difficulty.
C = Used only in special circumstances, if ever.
Standard design chart for HDPE
- Recommended wall thickness/length of flow, mm
Minimum __________ for _____________ distance
Maximum __________ for _____________ distance
Ideal __________ for _____________ distance
- Allowable wall thickness variation, % of nominal wall 10%
Outside: Minimum 150% w Maximum 175% w
Inside: Minimum 50% w Maximum 75% w
Maximum thickness 80% w
Maximum height 24% w
Sink marks: Yes X No_____
Maximum thickness 80% w
Maximum weight 160% w
Sink marks: Yes X No_____
Outside: Yes_____No X
Inside: Yes X No______
- Hollow bosses: Yes X NO________
Outside: Minimum 1°/side Ideal 2°/side
Inside: Minimum 1°/side Ideal 2°/side
No_____
Length: Minimum_____Maximum_____Process_____
Width: Minimum_____Maximum_____Process_____
- Special process- and materials-related design details
Blow-up ratio (blow molding) 1:1 to 3:1
Core L/D (injection and compression molding) 1:1 to 10:1
Depth of draw (thermoforning) 1.4 to 1.5:1
Other_______________
The reinforcing material most commonly added to HDPE is glass fiber. (Glass fiber is considered to be a reinforcing material while talc, calcium carbonate, and mica are considered to be fillers because there is more strength gained per pound of glass fiber than per pound of talc, calcium carbonate, or mica.) Glass-fiber-filled HDPE has excellent stiffness and higher temperature service than unreinforced HDPE. The chief drawbacks to using glass fiber in HDPE are increased cost and the abrasiveness of glass to the fabrication equipment. With the cost of surface-treated glass fibers being over $1 per pound, an HDPE so reinforced is much more expensive than unreinforced HDPE.
In deciding whether or not to add a filler or reinforcing agent to HDPE, the designer should consider the following criteria.
- Is there a property requirement in the application that is not met by natural HDPE?
- Can the requirement be met by adding a filler or reinforcing agent?
- Can the application support the additional cost of the filled system?
- Can another polymer (other than HDPE) offer the required properties in an unfilled state as economically as a filled HDPE system?
RESIN FORMS
HDPE can be purchased from resin suppliers in pellet, granular, or powder form. The most common mode of shipment is by bulk railcar. Bulk truck, 1000-1b and 500-kg cartons, and 50-1b and 25-kg bags shipments also are used. In quantities less than truckload, HDPE is generally sold by brokers or resellers rather than by resin manufacturers.
SPECIFICATION OF PROPERTIES
Master Outline of Materials Properties
Material HDPE
Property |
Test Method |
English Units |
Value |
Metric Units |
Value |
Mechanical |
|
|
|
|
|
Density |
D792 |
1b/ft3 |
58.7-60.3 |
g/cm3 |
0.941-0.967 |
Tensile Strength |
D638 |
psi |
2700-4400 |
MPa |
18.6-30.3 |
Tensile Modulus |
D638 |
psi |
|
Mpa |
|
Flexural Modulus |
D790 |
psi |
100,000-240,000 |
Mpa |
689-1654 |
Elongation To Break |
D638 |
% |
100-1000 |
% |
100-1000 |
Notched Izod At Room Temp |
D256 |
ft-lb/in. |
0.5-3.0 |
J/m |
27-160 |
Hardness |
D785 |
|
|
|
|
Thermal |
|
|
|
|
|
Deflection T@ 264 Psi |
D648 |
°F |
|
°C |
|
Deflection T@ 66 Psi |
D648 |
°F |
150-200 |
°C |
65-93 |
Vicat Softening Point |
D1525 |
°F |
248-266 |
°C |
120-130 |
UL Temp Index |
UL 746 B |
°F |
|
°C |
|
UL Flammability Code Rating |
UL 94 |
Linear Coefficient Thermal Expansion |
D696 |
in/in/°F |
60-110 Ã- 10-6 |
mm/mm/°C |
108-198 Ã- 10-6 |
Environmental |
|
|
|
|
|
Water Absorption 24 Hour |
D570 |
% |
|
% |
|
Clarity |
D1003 |
% Transmission |
|
% Transmission |
|
Outdoor Weathering |
D1435 |
% |
|
% |
|
FDA Approval |
|
|
Yes |
|
Yes |
Chemical Resistance To: |
|
|
|
|
|
Weak Acid |
D543 |
|
Not attacked |
|
Not attacked |
Strong Acid |
D543 |
|
Minimally attacked |
|
Minimally attacked |
Weak Alkali |
D543 |
|
Not attacked |
|
Not attacked |
Strong Alkali |
D543 |
|
Not attacked |
|
Not attacked |
Low Molecular Weight |
D543 |
|
Minimally attacked |
|
Minimally attacked |
Solvents |
|
|
|
|
|
Alcohols |
D543 |
|
Not attacked |
|
Not attacked |
Electrical |
|
|
|
|
|
Dielectric Strength |
D149 |
V/mil |
400-600 |
kV/mm |
15.7-23.6 |
Dielectric Constant |
D150 |
10(6) Hertz |
2.2-3.0 |
|
2.2-3.0 |
Power Factor |
D150 |
10(6) |
0.00005-0.003 |
|
0.00005-0.003 |
Other |
|
|
|
|
|
Melting Point |
D3418 |
°F |
|
°C |
|
Glass Transition Temp. |
D3418 |
°F |
|
°C |
|
PROCESSING REQUIREMENTS
Unfilled, unreinforced HDPE is not hygroscopic and normally does not require drying prior to processing. Filled HDPE will absorb small quantities of moisture and thus may require drying. If the HDPE is stored in outdoor silos, extreme temperature changes can cause moisture to condense on the pellets and thus necessitate drying. HDPE resins should be protected from contamination from dust, fibers, and other polymers as this type of contamination can affect both processing and end-use performance.
Normal processing temperatures for HDPE range from 350-600°F (177-316°C). The thermal stability of HDPE is usually good over the temperature range if the higher-temperature exposure times are kept relatively short; otherwise, the resin can degrade by cross-linking, chain scission, or both. It is normal for a slight change in melt index to occur during processing of HDPE.
Articles fabricated from HDPE can be ground and recycled. The recycled HDPE is normally blended with virgin HDPE before reuse, typically in amounts of 10% or less regrind. Above 10 percent, the physical properties of the fabricated product can be adversely affected. For best results, regrind must be protected from contamination from other polymers, dirt, paper, and so on.
PROCESSING-SENSITIVE END PROPERTIES
The impact strength, stiffness, and thermal stability of articles fabricated from HDPE are profoundly affected by processing conditions. For example, unidirectional orientation or overpacking a mold can greatly diminish the functional impact strength. If the processing temperatures are kept high and the process has a long resin residence time, the thermal stability of the finished article can be greatly reduced. The cooling rate will affect the stiffness and impact strength of the article: with a slower cooling rate, stiffness increases and impact strength decreases.
SHRINKAGE
Mold Shrinkage Characteristics
Mold Shrinkage Characteristics (in./in. or mm/mm)
Material HDPE
In the Direction of Flow
Thickness
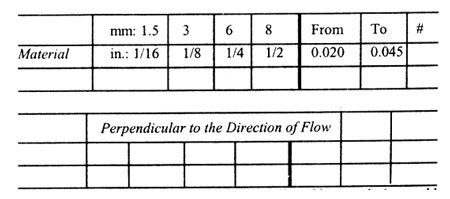
Note: Mold shrinkage is approximate. It is affected by part design, mold temperature, thickness, injection pressure, packing time, cycle time, orientation, gate design, gate size, gate location, glass content, glass size, and filler content.
Polybutylene (PB)
STRUCTURE
The name polybutylene (PB) refers to commercial semicrystalline resins based on high-molecular-weight isotactic poly(l-butene) homopolymer and copolymer. The chemical structure of poly(l-butene) is represented by:
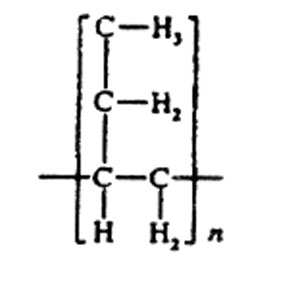
CATEGORY
PB resins are thermoplastic. They are polyolefins and exhibit strength and toughness in both rigid and flexible end-product forms.
HISTORY
Isotactic poly(l-butene) was first synthesized by G. Natta in 1954. Chemische Werke Huels of West Germany started the first commercial production in 1969 and discontinued the operation in 1973. In 1975, Witco Chemical Corp. built a PB plant in Taft, La., which was acquired by Shell Chemical in 1977. Pipe was the first important application of polybutylene and remains to this day as the dominant end-use market for PB. Shell Chemical is the sole U.S. producer of polybutylene.
POLYMERIZATION
PB is produced via stereospecific Ziegler-Natta polymerization of 1-butene monomer. Commercial products are based on isotactic high-molecular-weight polymer.
PROPERTIES
PB resins retain their good mechanical properties at elevated temperatures. Recommended upper and lower use temperatures are approximately 230°F (110°C) and - 4°F (-20°C), respectively. Pipe resins, specifically, exhibit excellent flexibility and resistance to creep, environmental stress cracking, chemicals, and wet abrasion. Plumbing code approvals and listings have been received from most states and cities, as well as from major regulatory and standards-making bodies.
Film grades of PB provide good tear and puncture resistance as well as high impact and tensile strength.
Glass reinforcement of polybutylene significantly increases its flexural modulus; it decreases its tensile strength and elongation. For a typical 40 wt% glass-filled pipe-grade PB, tensile properties at break are as follows: tensile strength, 1926 psi (13.3 MPa); elongation 14%; and flexural modulus (1% secant), 258,090 psi (1780 MPa).
APPLICATIONS
As earlier noted, the main commercial application for PB resins is in pipe. PB pipe has received hydrostatic design stress rating of 1000 psi (6.90 MPa) at 73°F (23°C) and 500 psi (3.45 MPa) at 180°F (82°C) from the Plastics Pipe Institute. Applications for small-diameter PB pipe include cold- and hot-water plumbing, the latter including residential and solar plumbing, and underfloor and hydronic heating. Other applications are in well piping, heat-pump piping, fire-sprinkler piping, and specialty hosing. Large-diameter PB pipe finds uses in the transportation of abrasive or corrosive materials at high temperatures in the mining, chemical, and power-generation industries.
Film applications of PB include heavy-duty shipping containers; food, meat and agricultural packaging; compression wraps; hot-fill containers; and industrial sheeting. Other applications include injection molding components, such as fittings for use in conjunction with PB pipe, and compression molding items, such as sheet for abrasion-resistant liner.
ADVANTAGES AND DISADVANTAGES
The advantages of PB in pipe end uses are its flexibility, toughness, and resistance to creep, environmental stress cracking, wet abrasion, and chemicals. PB films demonstrate high resistance to tear, puncture, and impact, and have high tensile strength.
The limitations of PB are a slower processing rate compared to other polyolefins in the heat state, a polymorphic transformation after melt processing, and low temperature brittleness.
PROCESSING TECHNIQUES
PB pipe can be fabricated via conventional single-screw extrusion technology using vacuum or pressure sizing for dimension control. After it exits from the die, the pipe should be cooled by water. The pipes can be joined via thermal fusion or mechanical fittings of several types.
The blown-film process is commonly used for producing PB film; PB film can also be cast on chill rolls. The films are heat-sealable. In addition, PB film can be coextruded or laminated with other films for specific applications. In-line embossing, with good pattern retention, is feasible without the application of heat.
Master Material Outline
Processability of Polybutylene
|
Process |
A |
B |
C |
Injection Molding |
X |
|
|
Extrusion |
X |
|
|
Thermoforming |
|
|
X |
Foam Molding |
|
X |
|
DIP. Slush Molding |
|
|
|
Rotational Molding |
X |
|
|
Powder Fluidized Bed Coating |
|
|
X |
Reinforced Thermoset Molding |
|
|
|
Compression/Transfer Molding |
|
X |
|
Reaction Injection Molding (RIM) |
|
|
|
Mechanical Forming |
|
X |
|
Casting |
|
X |
|
Flame Spraying |
X |
|
|
A = Common processing technique.
B = Technique possible with difficulty.
C = Used only in special circumstances, if ever.
RESIN FORMS
PB resins are produced in pellet form. In addition to pipe and film grades, homopolymer and copolymer general-purpose grades are offered.
SPECIFICATION OF PROPERTIES
Five crystalline forms of poly(l-butene) have been reported. In conventional melt processing, crystallization of the resins initially produces the metasable form II, which transforms to the stable form I over a period of 5 to 7 days at ambient temperature and pressure During the transformation, density, crystallinity, hardness, rigidity, stiffness, and tensile yield strength all increase to values characteristic of form I. After fabrication and transformation to form I, these resins show crystallinity of 48 to 55% and density of 0.93 to 0.94 g/cm.
Master Outline of Material Properties
Material Polybutylene (Pipe grade, nominal MI - 0.4 g/10 min)
|
Property |
Test Method |
English Units |
Value |
Metric Units |
Value |
Mechanical |
|
|
|
|
|
|
Density |
D792 |
lb/ft3 |
58-59 |
g/cm3 |
0.93-0.94 |
Tensile Strength |
D638 |
psi |
4640-5075 |
MPa |
32-35 |
Tensile Modulus |
D638 |
psi |
42,050-42,775 |
MPa |
290-295 |
Flexural Modulus |
D790 |
psi |
54,375-55,100 |
MPa |
375-380 |
Elongation To Break |
D638 |
% |
275-300 |
% |
275-300 |
Notched Izod At Room Temp. |
D256 |
ft-lb/in. |
12-15 |
J/m |
640-800 |
Hardness |
D785 |
Shore D |
60 |
Shore D |
60 |
Thermal |
|
|
|
|
|
Deflection T@ 264 Pa |
D648 |
°F |
129-140 |
°C |
54-60 |
Deflection T@ 66 Pa |
D648 |
°F |
216-235 |
°C |
102-113 |
Vicat Softening Point |
D1525 |
°F |
234-237 |
°C |
112-114 |
UL Temp Index |
UL746B |
°F |
|
°C |
|
UL Flammability Code Rating |
UL94 |
|
HB |
|
HB |
Linear Coefficient Thermal Expansion |
D696 |
in./in.°F |
0.003 073 |
mm/mm/°C |
0.000 013 |
Environmental |
|
|
|
|
|
Water Absorption 24 Hour |
D570 |
% |
[ 0.03 |
% |
[ 0.03[/TD] |
Clarity |
D1003 |
% Transmission |
|
% Transmission |
|
Outdoor Weathering |
D1435 |
% |
Black pigmented grades are weatherable |
% |
Black pigmented grades areweatherable |
FDA Approval |
|
|
Yes |
|
Yes |
Chemical Resistance To: |
|
|
|
|
|
Weak Acid |
D543 |
|
Not atacked |
|
Not attacked |
Strong Acid |
D543 |
|
Minimal to badly attacked |
|
Minimal to badly attacked |
Weak Alkali |
D543 |
|
Not attacked |
|
Not attacked |
Strong Alkali |
D543 |
|
Not attacked |
|
Not attacked |
Low Molecular Weight Solvents |
D543 |
|
Depends on solvent and temperature |
|
Depends on solvent and temperature |
Alcohols |
D543 |
|
Not attacked |
|
Not attacked |
Electrical |
|
|
|
|
Dielectric Strength |
D149 |
V/mil |
|
kV/mm |
|
Dielectric Constant |
D150 |
10(6)Hertz |
2.2 |
|
2.5 |
Power Factor |
D150 |
10(6)Hertz |
0.000 5 |
|
0.000 5 |
Other |
|
|
|
|
|
Melting Point |
D3418 |
°F |
265-266 |
°C |
120-124 |
Glass Transition Temp. |
D3418 |
°F |
-4 to -13 |
°C |
-20 to -25 |
After fabrication and transformation, the film-grade resins show 46 to 52% crystallinity and 0.90 to 0.92 g/cm3 density.
Typical properties of PB pipe resins are listed in the Master Outline of Materials Properties.
PROCESSING REQUIREMENTS
When PB is processed, the amount of regrind should not exceed 30 % of the input.
Vacuum or pressure sizing is required for dimensional control in single-screw extrusion of pipe.
PROCESSING-SENSITIVE END PROPERTIES
Increasing pipe extrusion speed or drawdown reduces the tensile elongation to break, a key property for quality control of PB pipe. The effects of blown film processing parameters on mechanical properties can be found elsewhere.
SHRINKAGE
Mold Shrinkage Characteristies.
Mold Shrinkage Characteristics (in./in. or mm/mm)
Material Polybutylene
In the Direction of Flow
Thickness
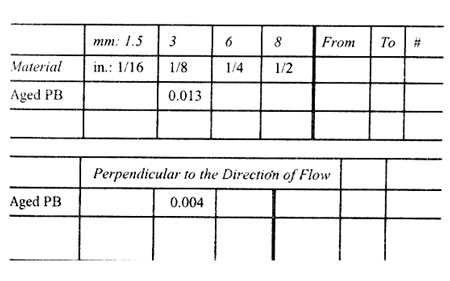
Note: Mold shrinkage is approximate. It is affected by part design, mold temperature, thickness, injection pressure, packingtime, cycle time, orientation, gate design, gate size, gate location, glass content, glass size, and filler content.
Fillers, Mica
CATEGORY
Mica is a reinforcing filler. In some resin systems, it reduces resin costs by as much as a third, but imparts certain selected property improvements reported to surpass those of traditional reinforcements that cost three times as much.
To do this, mica must meet requirements pertaining to size, shape, and, to some degree, composition.
There are two physically occuring categories of mica, "sheet" and "ground." Sheet mica is not used for composites. It is a relatively rare electrical grade, mined and sorted largely by hand. Ground mica, which is used as a reinforcing filler, is a processed flake of uniform size and thickness, generally recovered from pegmatite deposits.
SOURCE
Mica is part of the mineral class phyllosilicates, which is distinguished by a nearly complete basal cleavage between stacks of two-dimensional silicate crystals and a bond between negatively charged flakes linked by positively charged alkali ions. The layers slide, and thus, mica is "slippery." In making further distinctions between these mica silicates, the various deposits might be classed as muscovite (primarily potassium aluminum silicate), phlogopite (generally magnesium potassium aluminum-ion silicate), biotite (similar to phlogopite but of weaker crystal structure), and rarer compositions that include lepidolite and zinwaidite.
Typical function formulas of the more common micas are Muscovite, KAl2(A1 Si3O10)(OH)2; Phlogopite, KMg3 (Al Si3O10)(OH)2; and Biotite, K(MgFe)3(Al Si3O10)(OH)2.
Muscovite flake mica used in resin composites occurs very commonly in granitic rock deposits or metamorphosed schists, or gneisses, in North America. These deposits frequently occur concurrently with deposits of feldspar or spogamene in surface outcrop. Phlogopite mica occurs in metamorphosed sediments and is generally associated with pyroxenite. Su-zorite mica is a phlogopite that is characterized as having little associated quartz or silica.
Such ore bodies can be mined and harvested by open-pit surface operations. They are shallow in depth: less than 500 ft (152 m). Since mica ore usually occurs with weak horizontal bonds, it lends itself to ripping or recovery by bulldozer or power shovel with minimal blasting.
After the ore has been quarried, it is crushed to a uniform size having individual lumps small enough to feed processing operations uniformly but large enough to minimize retained water from snow or rainfall.
Mica beneficiation is designed to eliminate gangue mineral, reduce particles to fractions of uniform size, and delaminate the flakes into flat, laminar, thin entities. The properties of mica are somewhat in conflict with processing and tend to make it difficult to meet beneficiation objectives.
Ground mica is either wet ground, dry ground, or fluid energy ground. In some cases two processes are employed.
Wet grinding is inefficient and is rarely used for resin composites because of its high cost, limited availability, and narrow range of diameters and aspect ratios.
Dry grinding consists of processes that crush, screen, delaminate, and separate by air or gravity the mica in various stages and sequences suited to the ore used and the desired finished product. The initial stage includes two or three crushing steps, incorporating jaw crushers and hammer mills. These are followed by a closed circuit system of air separators, screens, and mills. In the case of Suzorite mica, special delaminating mills and gangue removal flotation devices are employed.
KEY PROPERTIES
Muscovites and phlogopites are the only types of mica of importance in resin composites. These types have many similarities as well as some significant differences.
All micas are highly resistant to oxidation, acids or alkalies, fire or heat, electrical energy, water, or organic solvents. Table 1 gives properties in which phlogopites and muscovites are similar and dissimilar.
There are differences that are often significant in plastics. Among these are the color, wide variation in decomposition temperature, water content, and stiffness. In general, the phlogopite micas have greater strength and high temperature resistance, however, the muscovites are slightly lighter in color. As a general rule, phlogopite composites tend to be tan or golden brown: muscovites are grey or beige.
Although there are several producers of mica in the United States, relatively few produce grades for plastics composite. They are listed in Table 2.
PROCESSING CHARACTERISTICS
|
Processing Property |
Phlogopite Mica at 40 wt% |
Viscosity |
Increased |
Melt flow |
Decreased |
Compounding |
Easier to compound compared to glass fiber or calcium carbonate |
Temperature |
Increased |
Injection pressure |
Slightly higher |
Flow in mold |
Difficult compared to virgin material |
Mold shrinkage |
Decreased |
APPLICATIONS
Depending on the individually varying cost/performance relationships, these benefits usually result from incorporating mica in composites:
Compared to all reinforcing fillers, mica provides
- Increased flexural strength, reduced gas permeability.
- Increased stiffness and reduced warp.
Properties of Phlogopites and Muscovites
|
Property |
Phlogopite |
Muscovite |
|
Similar |
|
Specific gravity |
2.7-3.1 |
2.7-3.1 |
Hardness, mohs |
2.5-3.0 |
2.5-3.0 |
Thermal conductivity perpendicular to cleavage, cal/cm |
16 Ã- 10(4) |
16 Ã- 10(4) |
Modulus of elasticity, psi |
25 Ã- 10(6) |
25 Ã- 10(6) |
(MPa) |
(172,375) |
(172,375) |
Tensile strength, psi |
38-42 Ã- l0(3) |
38-42 Ã- 10(3) |
(MPa) |
(262-290) |
(262-290) |
Specific heat at 77°F (250°C) |
0.20-0.21 |
0.20-0.21 |
|
Dissimilar |
|
Refractive index |
1.57-1.60 |
1.55-1.57 |
Maximum temperature with little decomposition °F (°C) |
500 (932) |
980 (1796) |
Coefficient of thermal expansion perpendicular to cleavage, °C |
1-2 Ã- 10(-5) |
11 Ã- 10(-6) |
Parallel to cleavage, °C |
14 Ã- 10(-6) |
10 Ã- 10(-6) |
Water of constitution. % |
1.0-3.2 |
4-5 |
Flexibility |
More |
Less |
Transparency |
Less |
More |
Compared to some reinforcing fillers, mica provides
- Increased heat-distortion temperature.
- Reduced cost and improved creep, and, where useful, such added benefits as improved ultraviolet and infrared resistance, microwave permeability, and heat shielding.
Mica provides little or no advantage over some lower cost fillers with respect to impact resistance, weld-line strength, and, sometimes, color. These drawbacks may be offset by combining mica with other fillers, modifying the resin, adding pigment, or using other additives.
Producers of Various Grades of Mica
|
Supplier |
Trade Name |
Type |
Grades Available |
J. M. Huber Corp. |
Aspra Flex |
Muscovite, dry/set ground |
4 |
Unamin Corp. |
Mica |
Muscovite, dry ground |
3 |
Franklin Mineral |
Alsibrone |
Muscovite, dry/set ground |
4 |
M.I.C.A. |
Mica |
Muscovite, dry ground |
2 |
Eagle Quality |
Micaflex |
Muscovite, dry |
2 |
Suzorite Mica Products |
Suzorite |
Phlogopite, dry |
19 |
(a) Formerly Martin Marietta Crop.
Physical Properties of Mica-reinforced Polypropylene Polymers
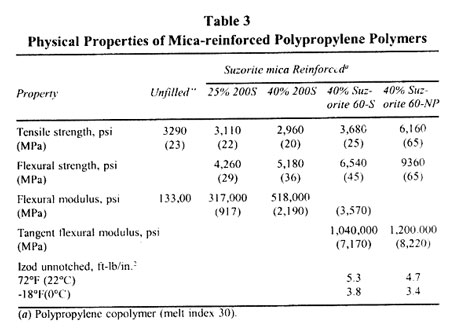
Physical Properties of Mica-reinforced High Density Polythylene Polymers
|
|
Unfilled(a) |
Suzorite mica 10% Glass |
Rein Forced(a) 10% 200 NP |
Unflled(b) |
Suzorite mcia 20% 200 NP |
Rein forced(b) 40% 200 NP |
Tensile strength, psi |
3,400 |
3,790 |
3,835 |
3,180 |
4,290 |
5,000 |
(MPa) |
(24) |
(26) |
(27) |
(22) |
(30) |
(35) |
Flexural modulus, psi |
131,000 |
207,000 |
195,000 |
123,000 |
330,000 |
670,000 |
(MPa) |
(903) |
(1,430) |
(1,350) |
(848) |
(2,280) |
(4,620) |
Flexural strength, psi |
2,070 |
3,750 |
5,850 |
(MPa) |
|
|
|
(14) |
(26) |
(40) |
Izod unnotched 72ºF (22ºC) ft-lb/'in.(2) |
|
|
|
No break |
13 |
6 |
(J/m) |
|
|
|
(690) |
(320) |
-18ºF (0ºC) ft-lb/in.(2) |
|
|
|
No break |
11 |
5.4 |
(J/m) |
|
|
|
(590) |
(290) |
(a) High molecular weight-high density polyethylene.
(b) High density polyethylene copolymer.
Physical Properties of Mica-reinforced ABS and calcium Carbonate-filled PVC
|
|
ABS + 5% Long Glass Fiber |
ABS + 10% Suzoritea mica (92%, 44 Microns) |
PVC + 10% CaCO(3) |
PVC + 10% CaCO(3) + 33.3% Suzorex mica 200-PO |
Tensile strength, psi |
7,930 |
7,480 |
|
|
(MPa) |
(55) |
(52) |
|
|
Flexural strength, psi |
10,300 |
11,000 |
7,060 |
8,950 |
(MPa) |
(71) |
(76) |
(49) |
(62) |
Flexural modulus, psi |
437,000 |
619,000 |
464,000 |
1,460.000 |
(MPa) |
(3,010) |
(4,270) |
(3,200) |
(10.100) |
Notched Izod. ft-lb/in. |
5.8 |
4.1 |
|
|
(J/m) |
(310) |
(220) |
|
|
Coefficient of thermal expansionb |
|
|
|
|
32-140°F, °F Ã- 10(-5) |
|
|
3.69 |
1.37 |
0-60°C, (°C Ã- 10-5) |
|
|
(6.65) |
(2.47) |
(a) Note the cquivalant performance of both materials
(b) The coefficient of expansion of mica-reinforced PVC is the same as aluminum.
Mica has been developed to perform well with polyolefins (chiefly polypropylene, polyethylene, and copolymers), thermoplastic polyesters, nylon, and urethanes. It shows promise with styrenics, PVC, and thermosets.
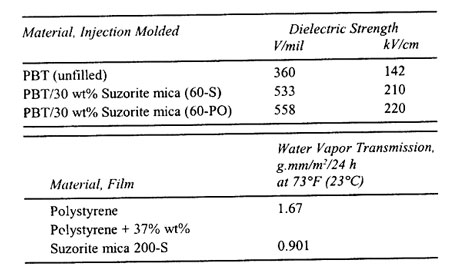
POLYMERS FILLED
Physical Properties of Mica-reinforced Nylon 6, 6
|
Property |
Unfilled |
40% Suzorite mica 325-PO Reinforced |
Tensile strength, psi |
11,300 |
12,50 |
dry (MPa) |
(78) |
(86) |
wet-16 h, 50°C psi |
8,460 |
10,400 |
(MPa) |
(58) |
(72) |
Flexural strength, psi |
12,300 |
20,000 |
dry (MPa) |
(85) |
(138) |
wet psi |
|
14,700 |
(MPa) |
|
(101) |
Flexural modulus, psi |
443,000 |
1,360,000 |
dry (MPa) |
(3,060) |
(9,380) |
wet psi |
126,000 |
840,000 |
(MPa) |
(869) |
(5,790) |
Izod notched, ft-lb/in. |
0.8 |
0.53 |
dry (J/m) |
(43) |
(28) |
wet ft-lb/in. |
|
0.93 |
(J/m) |
(50) |
Heat deflection, °F |
153 |
401 |
264 psi (1.82 MPa)(°C) |
(67) |
(201) |
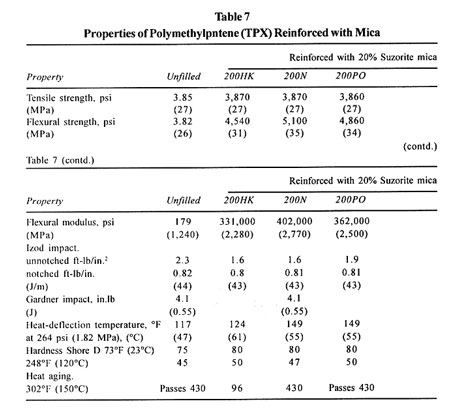
COMMERCIAL GRADES
The average bulk density for the products given is between 11 and 18 lb/ft3. The aspect ratio varies between 46 and 85, depending on the grade. There are other grades for commodity uses. They are sometimes tried for composites but generally are not recommended because of the low-purity mica aspect ratio. In the case of Suzorite mica, these are identified as Z grades (for oil-well drilling) and 80SF (a joint cement grade). Other producers have similar identifying grade nomenclatures.
The use of mica as a reinforcing filler will generally provide a reduction in cost, compared to the unfilled resin, on both a weight and a volume basis. Further, this unit cost will frequently be lower than other reinforcements providing similar properties. This latter distinction is important. Use of mica reinforcement is only economically prudent when the propeties sought favor the selection-in order words, for an "engineered" system, but not a general-purpose application.

Table
Advantages and Limitations of Various Filled Polymers
..
|
Polymer |
Advantages and Limitations |
|
Thermoplastics |
Polyolefins |
Improved flexural strength, dimensional stability, thermal expansion |
Thermoplastic polyesters |
heat-distortion temperature, ultraviolet stability, gas and liquid permeability |
Nylon Polystyrene |
Chemical resistance |
PVC |
Reduced impact strength; tensile strength is usually unchanged. |
Polyethersulfone |
Thermosets |
Polyesters |
Properties similar to thermoplastics, but increases viscosity and decreases |
Phenolics |
impact strength. Mica is usually supplemented with glass fiber |
Epoxies |
Reinforced Reaction Injection Molding (RRIM) |
Urethanes |
Improves nucleations but limited in some reinforcement properties |
COMPOSITE CHARACTERISTICS
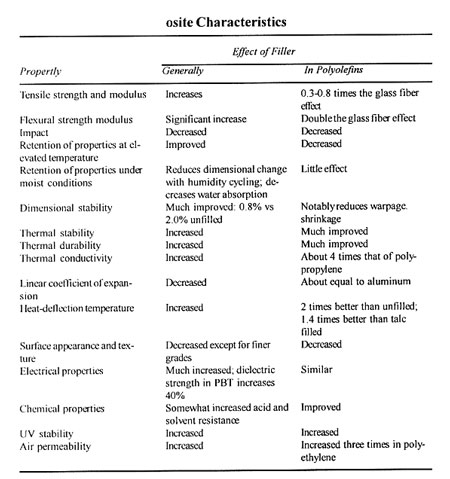
Colorants
INTRODUCTION
Color and its Measurements
The perception of color is dependent upon the observer's eye, brain, light and the object itself. Portraying a piece of plastic relates to its shape, size, gloss, opacity, surface structure and color.
Color is a personal sensation that is perceived differently by each individual. The stimulus producing the color response is light. One of the essential characteristics of a pigment is that it provides a certain color when illuminated.
Hue, lightness, and saturation are usually sufficient to describe the color of an object. Hue refers to the dominant wavelength of the color such as purple, red, yellow except for black, white, and gray. Lightness is a description of the amount of light reflected by a colored object as compared to a black to white scale. Light colors reflect more light and appear clearer and brighter than dark colors under the same illumination. Saturation simply refers to the strength of a particular color.
The wavelengths of the electromagnetic spectrum from dark red to violet are the ones that give the sensation of color. A beam of white light can be refracted by a prism into its constituent colors and can be recombined to produce white light. However, white light can be produced in addition to all other colors from the three primary colors, red, green, and violet.
The most common color vision defect is when the recognition of red, or green is diminished. Of the 8% of the population that suffers from some form of incurable color blindness, 95% are men. Color blindness is carried on the sex link character and is hereditary.
Since "normal" color vision is the average color vision of the population, then there is a range of color vision that is considered good. Two normal people looking at the same object may describe the color slightly different. When using a mechanical instrument to color match the impact is corrected by using a "standard observer" in the instrument.
Light
White light is sunlight reflected back from the sky whose wavelengths fall between 380 and 770 nanometers.
The ratio between the speed of light in a vacuum and its speed in a particular medium is known as the index of refraction of the medium. The greater the index of refraction the greater the degree the light is deflected when leaving or entering that medium. Dispersion is when a light beam of more than one frequency is split into a corresponding number of beams upon refraction. When light is refracted through a prism, the bank of colors that emerge are known as a spectrum. The colors of the spectrum are red, orange, yellow, green, blue, and violet.
Monochromatic light is rarely seen. When certain wavelengths are removed from white light, it appears to have a color. So the more continuous the spectrum of light the better the light simulates actual day light, and the more "natural" objects appear.
Because of the inconsistencies in natural daylight it is not advisable to do critical color matching by daylight. Daylight is affected by the seasons, time of day, weather, pollution and other elements in the environment. The best light for color matching plastics is a well-maintained standardized source of artificial daylight.
Fluorescent lamps are of different wavelengths than daylight and should not be used for color matching. Their only use is to know what the object will look like under fluorescent light.
When all the white light striking an object is reflected the object is white. A black object absorbs all the white light striking it and nothing is reflected back. The absorbed light is converted to heat. When light is differentially absorbed and reflected from an object it changes the spectrum reflected back causing color. For example, a yellow object absorbs all the wavelengths of light except those in the yellow spectrum which it reflects as yellow.
Fluorescence is absorbed radiant energy that is re-emitted at longer wavelengths, usually in the visible region. Optical brighteners are objects that absorb ultraviolet light and emit light in the blue region.
Scattering refers to the redirection of light. Scattered light is redirected through a medium so that a portion of it the light is traveling in all directions. The pigment and/or the plastic itself gives the object its degree of opacity. The scattering of light inside a plastic object which emerges is diffusely transmitted and has the color of the plastic.
An object in which there is no internal scattering yet transmits light is transparent. Unpigmented general purpose styrenics is an example. They appear as if one were looking through a glass. A translucent material is one in which there is a substantial scattering, resulting in a considerable amount of light being reflected. When no light is transmitted because there is so much scattering or because all the light is absorbed, the object is opaque.
Specular reflection occurs when light strikes a smooth surface and reflects it back like a mirror. Specular reflection leads to gloss. Specular reflection is the same color as the light source.
Colorants
Materials that give color and opacity to plastics are chemically characterized as either pigments or dyes. Pigments are finely pulverized natural or synthetic particles which may be of inorganic or organic origin and are insoluble in the matrix in which they are dispersed. Besides providing hue and hiding power, they can enhance chemical properties. The definition includes colorless extenders (i.e., calcium carbonate, and silicates). The significant difference between pigments and dyes is that dyes are soluble in the matrix in which they are dispersed, pigments are not.
Pigments containing carbon are organic; those without carbon are inorganic. The pigments used in coloring plastics are produced synthetically. Organic pigments are brighter, cleaner, and richer than their inorganic counterparts. However, they are also more easily affected by the destructive forces of heat, sunlight, chemical attack and migration, and they are more expensive. Performance characteristics, such as chemical and heat resistance, migration tendencies and lightfastness, are contingent upon its chemical composition. Chromophores are specific groups of atoms that determine a pigment's color.
Organic pigments that contain no inorganic constituents are known as toners. Maximum tinctorial strength is achieved when a toner is used. A lake is an organic pigment prepared by combining, extending, or diluting a toner with inorganic base. More than 90% of U.S. production of organic pigments are toners; the rest are lakes.
Inorganic pigments produce opaque dull colors. They have excellent resistance to heat, light, chemical aggression and migration. Typically, inorganic pigments have better exterior weathering durability and have higher densities than organic pigments.
PIGMENTS AND DYES
Major Organic Pigments
Permanent Red 2B is a mono azo pigment that is widely used in thermoplastics industry because it is inexpensive and has high tinting strength and good bleed resistance. The barium and calcium salts of Red 2B have higher color intensity but inferior lightfastness than the manganese salt derivative. However, the manganese salt accelerates the degradation of ABS, HIS, and polyethylene. The heat stability of this pigment is adequate for many applications.
Lithol Reds are frequently used because they produce sharp strong colors at a low cost in applications where stability is not critical. They are used frequently in PVC.
Quinacridones provide several shades of red that have good heat stability, good lightfastness and bleed resistance in vinyls, polyolefins and polyesters. However, in other systems (such as acrylics, nylons, and polystyrene, where the processing temperature range is high) only the violet and blue shade quinacridones can be used.
Carbozole dioxazine possesses a violet hue. It is used extensively in applications where excellent bleed and heat resistance are required along with good lightfastness. It is such a strong pigment that it is commonly used to redden phthalocyanine blue.
Perylene pigments are diimides of perylene tetracarboxylic acid that provide shades of red or maroon. They provide excellent resistance to chemicals, migration and light. Phthalocyanine green and blue are brilliant colors characterized by being very heat resistant and lightfast. They are the workhorses of the plastic industry. The crystal structure of phthalocyanine determines its color. Phthalocyanine blue has a shade range from red to peacock green, phthalocyanine green has a narrower range from blue to yellow shade. However, the green pigment is more stable than the blue. Shades of the blue pigment are subject to change due to crystallization whereas the green pigment's shades arise from halogenation with chlorine and bromine.
Diarylide yellows and oranges, however, are diazos that allow for many shades and a wide range of properties. Lightfastness, migration, and heat stability are a function of the chemistry involved. Diazo condensation pigments and tetrachorisoindolinones pigments are yellow pigments offering improved performance properties.
PY17 is used extensively in vinyls because of its strength and resistance to migration. HR yellow PY83 is a reddish-yellow that is highly transparent and has fair heat resistance.
Easily dispersed pyrazonone oranges have excellent heat and chemical resistance. They are used widely in polyethylene, polystyrene, vinyls, polyester, phenolics and polypropylene.
Perinone orange, PO43, is an expensive vat pigment that has excellent heat, light and migration resistance is widely used in vinyls.
Inorganic Pigments
Natural inorganic pigments are of mineral origin, mined in the chemical form in which they will be used. Synthetic inorganic pigments are manufactured from inorganic raw materials, making them finer than their natural counterparts. These finer particles are easier to disperse. Chemical resistance is a function of their chemical makeup. For example, iron blue pigments exhibit poor alkali resistance but ultramarine blue is highly stable towards alkalies but sensitive to acids.
Cadmium sulfide and blends of it with cadmium selenide provide a wide array of colors from greenish yellow through orange, red, and maroon. Cadmium sulfide is naturally golden yellow; selenium gives the red shades.
The cadmiums are easily dispersed and provide vivid colors. They are used extensively in thermoplastics because of their excellent resistance to heat and chemical attack. Although cadmium and selenium are considered toxic they are so inert that they can be used in all plastic applications not having direct contact with food.
Blends of cadmium sulfide with cadmium selenide and zinc sulfide (extended with barium sulfate up to 60% by weight) are how lithopone type cadmiums are supplied. This extension with barium sulfate improves dispersibility and economics.
Lead chromate pigments are economical inorganics that yield primrose, lemon yellow, medium yellow, and orange shades. A large range of yellow colors can be produced using these pigments. Although chromates yellow in the presence of atmospheric sulfides and are sensitive to acids and bases, surface treatment of the chromates has produced pigments that are more chemical resistant, heat resistant, and have improved lightfastness.
Another important lead-containing pigment is molybdate orange. This easy-dispersing pigment is often blended with other inorganics and organic pigments. The strong light orange to red colors provided by inexpensive molybdate pigments, coupled with technology that has improved their chemical and heat resistance makes them broadly applicable. Commercially available molybdate orange contains lead chromate, lead molybdate, and lead sulfate.
Cobalt blue is an aluminate that comes in shades from blue to turquoise. Although expensive and weak in tint strength, cobalt aluminate pigments have excellent heat and light stability and chemical resistance. Migration resistance and weatherability are also excellent. Such pigments generally are selected for applications in which the need for performance outweighs cost.
Sodium aluminum sulfosilicates are characterized by permanence, heat resistance, and alkaline stability. By removing sodium during production of this pigment the color shifts from blue towards a pink and violet, which is the commerical color range. They provide a lightly reddish blue pigment that is clean and vivid. However, poor acid resistance and tint strength restricts their use in plastics.
Chromium oxide green is a dull colorant with remarkable resistance to sunlight, heat, acids, and bases. This green pigment is capable of reflecting infrared lighting, making it a colorant of choice for military camouflage.
Chrome green, an altogether different pigment from chromium oxide green, is made by mixing chrome yellow with iron blue. Because this class of pigments is cheap and exhibits good hiding power, it is used in applications where good heat stability and lightfastness are not required. A typical plastic application is in unsaturated polyesters.
Synthetically produced inorganic white titanium dioxide is nontoxic and stable. TiO2 is widely used as an exteriors colorant because of its outstanding resistance to the destructive forces of sunlight. Two different crystal forms of TiO2 exist: anatase and rutile. These two species render different chemical and physical properties. Very fine-particle-size rutile TiO2 (0.2 to 0.35 microns in diameter) is used to provide high strength and blue tone. Superior weathering is a feature of rutile. Rutile also is responsible for giving opacity, brightness, and whiteness to plastics. However, the less abrasive anatase type is an excellent choice where resistance to chalking and ozone is important.
Carbon black is an organic pigment produced by the incomplete combustion of solid, liquid, or gaseous hydrocarbons. There are five classification of carbon blacks; namely, lampblack, channel black, thermal black, furnace black and acetylene black. Each of these blacks finds applications in different markets.
Carbon blacks require lots of energy to achieve a good dispersion, but they are low cost and perform as an excellent heat and light stabilizer. Around 250 shades of blackness or jetness are visible to the naked eye merely by going from the smallest particle size to the coarsest. Usually only 1% carbon black will produce a masstone black part. The major considerations in selecting a carbon black are particle size, structure, surface area, and pH.
Characteristics of Dyes
Dyes produce transparent effects with maximum strength and brilliance. Unlike pigments, dyes cannot protect the resin from ultraviolet-light degradation. However, many dyes are satisfactory in terms of their own lightfastness. When tints of the dyes are made, dye fading is more easily seen.
Dyes are candidates for coloring thermoplastics because of their good to excellent heat stability. Azo dyes are used widely in rigid PVC, styrenics, acrylics, styrene and phenolics because they are readily available, have good fastness qualities, and are economical. Anthraquinone dyes have been adapted as plastics colorants because of superior heat and light stability (even though they cost more). Dyes sensitive to heat and light, such as xanthenes, are used in acrylics, polystyrene, and rigid PVC because of their brilliance and fluorescence.
These three classes of dyes constitute about 60% of the commercial dyes available. The remaining types of interest in the plastic industry are azine, aminoketone, perinone. indigoid, triarylmethane, quinoline, monomethine, indophenol, phthalocyanine, and Fluorescent Brightener 61.
The foregoing information on pigments and dyes is meant to serve as a representation of major types used in plastics. Full details and specifications are beyond the scope of this article, but they can be found in the Colour Index (CI), a renowned publication of colorants that provides generic names for identifying dyes and pigments with respect to use, and numbers for identifying them according to chemical composition. This index provides comprehensive data on the performance characteristics, chemical profiles, and use.
Colorant Forms and Functions
There are six basic ways in which color is supplied, each with its own advantages and disadvantages.
- Dry color, as the name implies, is pigment added to resin for subsequent blending and processing applications.
- Direct compounding is an inventory-efficient technique by which the customer adds the pigment to the resin inplant, in quantities sufficient for a specific product run.
- Color concentrates are pellets containing a high level of pigment and are compounded directly into the resin in the molten state. The concentrate is then added to natural resin in predetermined rations dependent on desired color intensity in the finished product.
- Color pastes are pigments or dyes that are dispersed in a liquid vehicle (such as a plasticizer) compatible with the plastic resin. Pigment loadings of pastes are usually very high.
- Liquid colors are pigment dispersions in vehicles that possess universality with respect to the resin. These too are of a very high loading and are pumpable.
- Freeze-dried colors are pigments dispersed in a vehicle that solidifies at room temperature or when chilled. They are usually commercialized in flake or chip form.
Dry color can be a pigment or blends of pigments mixed (usually with a metallic stearate) to facilitate dispersion. Blends are sold in preweighed packets or in bulk quantities in pails. The processor adds dry color to the base resin and tumbles them in drums to thoroughly disperse the color/resin mixture to be fed into the hopper. The advantages of dry color are that large and small quantities are easily accessible at an economical cost. And because the colorant agent is 100% color, storage space is minimal.
Dry colors have some disadvantages. The dust they generate can be a health or cleanup problem. Dust can also contaminate other colors and/or manufacturing equipment. Cosmetic imperfections, such as streaking and specking, can occur when dry color is used; this is a result of dry pigment particles being agglomerated rather than being broken down as small as possible. When pigment particles are used in the agglomerated state their cost effectiveness as colorants is reduced. Finally, the time it takes to purge an injection molder to go from one dry color to another is longer than when color concentrate or dispersed color is used.
Concentrates are melt bends of resin and pigment mixed with a given weight of virgin carrier resin. The advantages of concentrates are ease of handling and color consistency from batch to batch. The negative aspects are that concentrates are relatively expensive and cannot be used with all resins, thereby increasing inventory needs. Also the lead time to fill orders can be lengthy, and the customer is generally required to purchase a minimum quantity of a special color. Metering systems for concentrates involve moderate to high capital expenditure.
Liquid colors provide a great variety of pigmentation possibilities for thermoplastic resins, including engineering grades. A simple yet extremely accurate peristaltic pump has been designed to meter liquid colors to the throat of the plasticating screw. This provides for very critical reproductions in color matching. Liquids require less downtime than any other system when cleaning or purging. A wide array of liquid colors is available and compatible with most thermoplastic resins-therefore reducing inventory and maximizing usable plant space. Once the system has been set up, virtually no supervision is needed.
The Importance of Dispersion
Dispersion, the procedure for producing color concentrates and liquid color, involves three distinct steps. Initial wetting, deagglomeration and air displacement are the prerequisites to disperse a pigment fully and develop it to its maximum potential.
Initial wetting involves blending the vehicle with the pigment. At this stage, the compatibility between pigment and vehicle is readily seen. If insufficient wetting is achieved the pigment may be poorly dispersed no matter how good the following dispersion steps are. Therefore, precise care must be taken in preparation of the premix.
Deagglomeration (size reduction) is the breaking up of clusters of individual pigment particles held together by surface attraction. The right amount of mechanical energy must be applied to isolate the pigment particles so they can be surrounded by vehicle. The mechanical energy required to deagglomerate a pigment is critical, since over-shearing can degrade the dispersion. In order to obtain maximum yield, it is necessary that the dispersion requirement of each pigment be evaluated.
Replacing the air on the pigment surface with vehicle is paramount in developing the color of pigments and is synonymous with intimate wetting. Unless the pigment particles are intimately wetted there is no shearing effect on the pigment aggregates when mechanical energy is dissipated into the liquid dispersion.
Color yield by a pigment dispersion is a function of particle size distribution. Large particles (15 microns) will have rough surfaces with streaking. Small particles (0.3 microns) give maximum intensity accompanied by high transparency. But moderate to small particles result in high hiding power and tinting strength. Measuring the effect of the pigment particles (not particle size) is the method by which to determine the degree of dispersion. The Hegman grind gauge is a common tool used to measure degrees of dispersion. Preparing tints (colors let down with TiO2) of colored dispersion is another route for determining the degree of dispersion if a control is available.
Master batching is another method of making quantities of a particular color in a particular resin. The larger the batch purchased, the greater the quantity of consistent-color plastic that can be achieved. However, this requires a lot of inventory and space. Usually it is not profitable to fill orders of the colorant in small batches. Special colors must be ordered in large quantities, and they sell for a premium.
Coloring Do's and Dont's
The compatibility of components used in a color system should be known or checked because unexpected problems may occur in production or product use. A liquid color's vehicle must be compatible with the resin. Otherwise the plastic may exude the vehicle. Oil-soluble dyes are not compatible with olefins. They bleed severely. Cadmium pigments are acid sensitive and should not be used with acidic pigments or else the cadmiums will degrade. Phthalocyanine blue causes HDPE to warp. Fluorescent pigments tend to solvate, so certain additives cause these pigments to lose their fluorescence. Red 2B yellows with the addition of zinc stearate. Chrome yellow pigments, which suffer from poor alkali resistance, cannot be used with alkaline extenders such as calcium carbonate.
When color matching in thermoplastic resins such as ABS, SAN, thermoplastic urethane and polycarbonate, it is necessary that these resins be dried before using. Otherwise, residual moisture will cause streaks resembling stress cracks in the finished article. Pigments used in urethane formulations must be dried so that the moisture does not interfere with the cure.
Exposure to outdoor light can dramatically alter color stability. Some pigments get darker, some fade, others change color entirely. The lightfastness of a color is dramatized when it is mixed with a white pigment or when a low-pigment-volume concentration is used.
Migration is the mobilization of some component of a plastic to the surface. There are four subcategories of migration or leaching that are known to occur. Bleeding describes diffusion of a colorant from its substrate to an adjacent object. Crocking is when the pigment may be removed from the surface of the plastic by rubbing it. After the removal of the color pigment by abrasive rubbing, the surface takes on an appearance of metallic bronze and is known as bronzing. Another condition known as plateout occurs when an undesirable film forms on the surface of the metal die or calendaring rolls during processing of the plastic.
Heat resistance of a pigment should be greater than that of the processing condition requirements of the plastic. The length of exposure of the pigment to high temperatures is critical when color matching. Pigment burnout occurs when the pigment becomes unstable for the processed time and temperature. Inorganic pigments perform much better in regard to heat resistance than the organics. Most organic pigments are stable for only a few minutes at a maximum of 450°F (232°C), the inorganics generally can be held for over 550°F (288°C) for 5 to 10 minutes.
For decorative effects where opacity is desired, it is important to use enough pigment to produce the desired effect. It is possible to use more pigment than is actually required to achieve opacity. This in turn affects the economics of the finished product. Generally, inorganics alone are sufficient to produce good opacity. But most organic pigments and dyes require opacifiers such as titanium dioxide in order to achieve good hiding power.
Toxicity is an important concern. The U.S. Food and Drug Administration (PDA), under the Federal Food, Drug and Cosmetic Act, makes certain that all the substances in colorants (pigments, vehicles and additives) are acceptable for incorporation into plastics intended for direct food contact. If no prior sanctions exist, the company desiring to use a particular color must apply for and receive approval under a lengthy and complex test protocol.
Occasionally, the ideal colorant for a system is cost-prohibitive and cannot be used. The formulator must then prepare a color match pooling experience and knowledge that will provide the user with all the properties needed to withstand the application's environment. Compromises must be made between seller and user regarding the colorant's performance properties.
Color Measurement and Matching
There are two basic ways of evaluating color: optical and mechanical.
Measuring and describing color can be performed either with a machine or person using a standard as a reference point. In either case catalogs with visual comparison of samples provide useful descriptions of color.
For example, the Munsell book of color contains over 1500 color chips in matte and glossy surfaces. In this systemized book the test swatch and the colored sample in the book (which has a neutral background) are laid side by side and compared in daylight. From this comparison any color can be accurately described by using the Munsell color notation. This notation describes the hue with a number ranging from 1 to 100 or with a letter or letters plus a number. The value scale indicating lightness is characterized by a number where 0 is black and 10 is white. The numbers 0-16 are used to describe the chroma, Munsell's term for saturation. Ten letters make up the hue circle, R, YR, Y, GY, G, BG, B PB. P, and RP which are each divided into 10 parts with some containing decimals such that 10G is the same as OBG. The system of characterizing hue by a number and letter is popularly used in many color notation systems.
The Universal Color Language is another system: it provides a description of colors at a variety of complexity levels. Level I has 13 adjectives describing the hue. Level II increases the accuracy by the addition of 16 more descriptive color adjectives. The Inter-Society Color Council-National Institute of Standards and Technology, which has 267 names for colors, is level III. Level IV contains the 1500 color samples from the Munsell Book of Color. At Level V, a comparison of a colored sample against the nearest color in the Book of Color is required. From the difference in colors from a visual judgment a new value is assigned to the color in question. This allows for roughly 100,000 different colors to be assigned a number. An instrument that measures color differences with mathematical expressions is necessary for Level VI, which allows a few million different colors to be characterized.
Color matching requires that a uniform way of describing color differences or tolerances be postulated. In plastics coloration, allowable tolerances be agreed upon in advance between manufacturer and purchaser. Computer readouts of color matches against a given control allows us to numerically assign a value of just noticeable differences (JND).
David MacAdam has proposed an equation for measuring color differences which is now widely used. Hugh Davidson and Fred Simon have modified this formula so that lightness is incorporated into the effect on color differences. These are called MacAdam units and are used throughout the plastic industry.
The International Commission on Illumination has founded a mathematical treatment of color data so that a common principle can be used in calculating color. The committee has established tristimulus values to describe color, calculated from the transmission values of a sample. The values are designated X, Y, and Z, which correspond to red, green, and blue respectively. Two samples that have the same tristimulus values are considered matches.
There is now a wide variety of instruments that provide an objective way of describing color. And the description of a color can be easily verified from lab to lab.
The spectrophotomer is used in color matching and it is capable of measuring a spectral reflectance curve. The price of these valuable instruments has decreased over the years, now making them affordable to just about any serious color-matching laboratory. Because the spectrophotometers were rather expensive years ago, some laboratories purchased tristimulus colorimeters. Although these colorimeters accurately measure color differences, they cannot measure spectral reflectance curves and subsequent tristimulus values.
Color matching involves a customer sending a sample to a coloring house and specifying how critical the match must be before it will buy the colorant.
The phenomenon of two objects being a color match under a certain light but looking different under another illuminant is called metamerism. If a color match is made using the same pigments and resin, the wavelengths reflected or absorbed are the same regardless of what illuminant is used. In this case, metamerism is eliminated. Preventing a metameric match is a primary consideration in custom color matching. Because one cannot visually see spectral reflectance curves it is necessary to use a spectrophotometer. Whenever the spectrophometric curve of the standard intersects with the curve of the sample a metameric condition exists. The conditions for producing a non-metameric match require that the same pigments and resin be used. The skilled color matcher can usually determine what pigments are present in the plastic.
Degree of dispersion is important in color matching because the color becomes fully developed only when it is properly dispersed. Maximized color development minimizes the chance of metamerism. A good pigment dispersion supply house can do the job. But color matching must always be performed in the same resin the customer is using. Otherwise the match may appear different and be judged unacceptable.