Rice
Production and Utilization
I. ORIGIN OF RICE
Rice (Oryza sativa L.) is one of the leading food crops of the world, and is produced in all continents. Rice was an important food even before the dawn of written history. One center of origin of cultivated rice is thought to have been in Southeast Asia, that is, in eastern India, Indo-China, and southern China. Another probably was in Africa.
The origin of cultivated rice has been studied by many investigators, and the results of these studies summarized. Most think that the original ancestral species no longer exist and that present varieties have evolved from known species. Most rice varieties currently in production are in the species O. sativa. However, some varieties grown in Africa are in the species O. glaberrima Steud.
The true number of species of the genus Oryza is not known. A large number have been described, but most authorities agree that there are 30 or fewer valid species.
II. COMPARISON OF RICE WITH OTHER CEREAL GRAINS
All of the principal cereal crops are used for food. Rice, wheat, and rye are used primarily as human food. Maize, sorghum, and millet are important foods in some countries but are generally considered to be feed crops. Barley and oats have important uses for human food and beverages but most of the production is used for feed.
The crop area and production of rice in the world are second only to wheat. The world rice crop area is only about 58% as much as that for wheat. However, the average yield of rice is much higher than for wheat, so that the total world production of rice is only about 6% less than for wheat. Over 95% of the world production of rice is used for human food.
Rice is generally considered to be a tropical crop. It is, however, an important crop in subtropical and temperate zones. The yields are higher in temperate areas than in the tropics.
In many Asian countries, the average individual gets half or more of his total caloric intake from rice.
III. COMPOSITION OF RICE AND CEREALS
The composition of brown rice; whole-grain wheat, maize sorghum, millet, and rye; lightly pearled barley; and dry oatmeal is shown in Table 2.1. Rice is comparatively high in caloric value, N-free extract, and niacin, and is comparatively low in protein although rice protein has a fairly good balance of the essential amino acids (Table 2.3). The fat content of brown rice is somewhat higher than in
Table 2.1. Average composition of cereal grains.
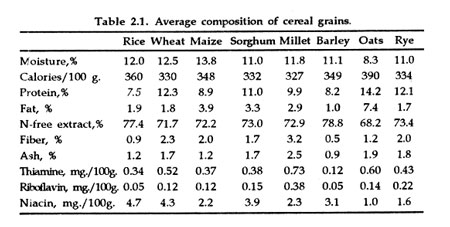
pearled barley, about the same as in whole-grain wheat, and lower than for other cereals. The fiber content is lower than for other cereals, except for pearled barley. The ash content is slightly higher than for pearled barley, about the same as for corn, and lower than for the other cereals. Brown rice is comparatively low in thiamine and riboflavin.
The composition of rice is influenced by variety and environment. In a study of eight varieties it was found that for the endosperm, ash content ranged from 0.45 to 0.50%; nitrogen, from 1.21 to 1.36%; lipids, from 0.41 to 0.61 %; and starch, from 89.6 to 90.7%, based on the varietal average of material grown at three locations for 3 years. In this same study, the average values at each location for ash ranged from 0.47 to 0.50%; nitrogen, from 1.20 to 1.41%; lipids, from 0.48 to 0.59%; and starch, from 89.3 to 91.0%.
Table 2.2 Composition of brown milled and parboiled rice
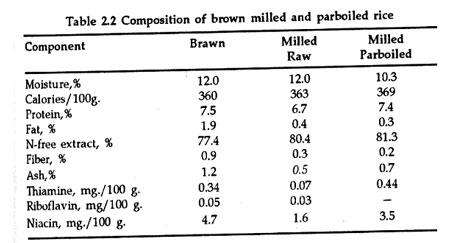
The composition of brown, milled raw, and milled parboiled rice is shown in Table 2.2. The principal difference between brown rice and milled raw rice is that the protein; fiber, thiamine, and niacin are higher in brown rice. Parboiled milled rice is similar to brown rice in protein, thiamine, and niacin content.
The amino acid composition of the protein in cereals is shown in Table 2.3. Among cereals, rice has a comparatively high content of essential amino acids and fairly low content of glutamic acid and some other nonessential amino acids. The nutritional level of rice is comparatively high among cereals and other grain and root crops. It was found that rice and rice by-products improved diets based on potatoes, sweet potatoes, corn meal, pinto beans, and cottonseed meal. The favorable amino acid balance of rice is shown by the fact that rice can be supplemented to bring the lysine content to an optimum level at lower cost than for wheat, corn, millet, or sorghum.
IV. BREEDING RICE VARIETIES WITH SPECIFIC PHYSICOCHEMICAL CHARACTERISTICS
Breeding efforts in recent years have been directed toward developing varieties with specific kernel chara-cteristics. Breeding programs have as a major objective the development of varieties that meet specific needs of processors and consumers. The techniques needed to test breeding lines for these characteristics.
Requirements of consumers and processors have been firmly established, so that all new varieties are checked carefully for conformity to the size, shape, and physico-chemical characteristics of the types they represent.
Consideration also is given to developing varieties with improved quality and quantity of grain protein content. There is a wide range in protein content among rice varieties. There also is evidence that protein differences are inherited. The progeny of crosses between varieties that differ in protein content have not been tested for yield, but preliminary observations indicate that it should be possible to develop varieties with higher protein without sacrificing their yielding ability.
Studies of amino acid content show varietal differences. In a comparison of 19 amino acids in 4 varieties, statistically significant differences were shown for 10 of the amino acids. For example, in one comparison the amount of lysine in one variety was only about 78% as high as another variety that was tested. No experimental results are available, however, on the heritability of differences in amino acid level.
V. INDUSTRIAL USES FOR RICE AND RICE BY-PRODUCTS
Rice is a fairly high-cost commodity, which limits its use in industrial products. However, rice-milling by-products have many uses.
Since hulls or husks make up about 20% of the rough rice as it is received at the mill, considerable effort has been made to find uses for them. At one time most of the hulls were used as fuel in the steam plants which provided power for rice mills, but this is no longer a common practice. At the present time hulls are used primarily in feeds, as an adjunct to prevent caking in fertilizers, for mixing with small-seeded forage plants for reseeding ranges, for animal and poultry bedding, and as loose insulating material in walls of buildings and cold-storage facilities. Finely ground hulls also are suitable for use in phenolic resins and in phenol-formaldehyde plywood glues.
Rice hulls are seldom used for production of methyl alcohol, acetic acid, and other products because cheaper sources are available. They are not suitable for making paper or rayon pulps because of their short fibers and relatively low alpha-cellulose content. Rice hulls are a good raw material for the manufacture of furfural but are not widely used for this purpose.
The total by-products of milling are sometimes utilized as "rice mill feed," which usually contains about 61% hulls, 35% bran, and 4% polish.
Rice bran is generally used in animal feeds, and its efficiency as a feed supplement has been widely tested. In one experiment it was found that rice bran can replace up to half the corn in a swine diet before the carcasses were classified as soft pork.
Rice polish, like rice bran, is rich in nutrients. Rice polish is used primarily for stock feed, but some is used in baby foods and health foods.
CARYOPSIS AND COMPOSITION OF RICE
I. INTRODUCTION
Rice grain is relatively less studied than the other major cereal grains: corn, barley, and wheat. In this chapter, emphasis is given to recent publications, since these cite earlier studies.
The rice grain (rough rice) consists of an edible portion, the rice caryopsis, and its covering structures, the hull or husk. The rice hull constitutes from 18 to 28% of the rough-rice weight.
II. GROSS STRUCTURE OF THE RICE CARYOPSIS AND ITS MILLING FRACTIONS
A. Gross Structure
The rice fruit is a caryopsis in which the single seed is fused with the wall of the ripened ovary (pericarp), forming a seed-like grain Fig. 2.1. The caryopsis is called brown rice because of its brownish pericarp. Red rice has a red pericarp or tegmen, or both. The tip of the caryopsis is somewhat oblique, corresponding to the size of the husk lemma, which is larger than the palea. The surface of the caryopsis has ridges corresponding to those of the lemma and palea.
The rice caryopsis varies widely among cultivars in shape and size, length and width. The rice is classified by length into sizes of extra long, more than 7 mm; long, 6.0 to 7.0 mm.; medium or middling, 5.0 to 5.9 mm.; and short, less than 5 mm. (30). The corresponding shape classifications for brown rice based on a length-to-width ratio are: slender, more than 3; medium, 2.4 to 3.0; bold, 2.0 to 2.39; and round, less than 2. Japonica cultivars generally are bold or coarse and short-grain, whereas indica cultivars are usually long- or medium-grain.
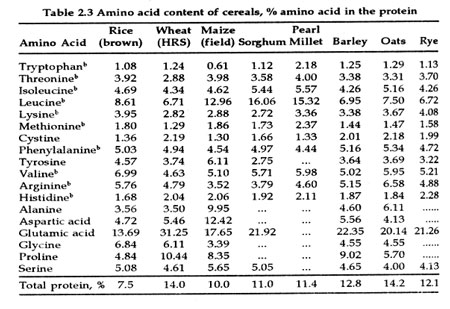
The weight distribution of the various parts of brown rice is: pericarp, 1 to 2%; aleurone plus testa, 4 to 6%; germ, 2 to 3%; and endosperm, 89 to 94%. In the germ fraction of brown rice, the weight distribution is epiblast, 0.26%; coleorhiza, 0.18%; plumule, 0.34%; radicle, 0.18%; and scutellum, 1.18 to 1.4% (56). A related study reported pericarp, testa, and aleurone, 6.5%; scutellum, 2.0 and 2.1%; embryo, 0.8 and 1.1%; and endosperm, 90.4 and 90.6%.
B. Pericarp and Tegmen
The caryopsis is enveloped by a fibrous pericarp varying in thickness. The pericarp consists of six layers, five of which are transversely elongated. The pericarp is further classified into outer epicarp, consisting of transversely elongated cells with deeply wavy end walls; hypoderm or mesocarp, also of transversely elongated cells but with straight walls; cross cells of transversely elongated vermiform cells which, except for their transverse arrangement, are quite like the cells of the next layer; and typical tube cells.
Of these layers, the epicarp, with the deeply sinuous end walls and arrangement of cells side by side in rows, is highly characterized in surface view. These cells may be distinct in some varieties but not in others. The cell-walls of the pericarp are 2m thick and absorb stains for protein, hemicellulose, and cellulose.
Next to the pericarp are two layers of cells representing the remains of the inner integuments, the tegmen or seed coat. These layers are denoted as the sepermodern and the perisperm. In surface view the two layers have delicate walls and the cells are side by side in rows, but the elongation is transverse. The perisperm cells are characterized by their beaded walls. The tegmen contains a fatty material.
C. Aleurone Layer
Both the endosperm and the embryo are enclosed by the aleurone, which lies beneath the tegmen. The aleurone may have from one to seven cell layers and is thicker on the dorsal than along the lateral and ventral surfaces. Cultivars differ in the thickness of their aleurone layers. Coarser or bold short grain cultivars tend to have more cell layers than slender long-grain cultivars.
The aleurone layer is composed of quadrangular or rectangular parenchyma cells with thin (2m) wall. These cells are filled with small, protein-rich aleurone grains enclosed in a sheath of fat-staining material. Within the aleurone layer at the dorsal side two or more short layers of polygonal parenchyma cells may occur, also containing aleurone grains. The cell-walls of the aleurone layer react positively to stains for. protein, hemicellulose, and cellulose. Upland cultivars have more aleurone cell layers on the dorsal and ventral sides than lowland cultivars. Warm temperatures during ripening also increase the number of aleurone cell layers, but on the dorsal side only.
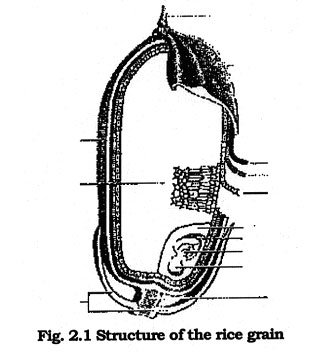
D. Embryo
The embryo or germ is extremely small and is located on the ventral side of the caryopsis. In a longitudinal section of the grain, the outline of the embryo appears more or less lenticular Fig. 2.2. It contains the embryonic leaves (plumule) and the embryonic primary root (radicle), which are joined by a very short stem (hypocotyl). The plumule is enclosed by a cylinder-like protective covering, the coleoptile, and the radicle is ensheathed by a mass of soft tissue, the coleorhiza. The embryonic axis is bound on the inner side by the scutellum (cotyledon), which lies next to the endosperm. The embryo is enclosed on the outer side by the aleurone layer. The coleoptile is surrounded by the scutellum and the epiblast, the vascular trace which is fused with the lateral parts of the scutellum.
In a longitudinal section, the plumule and radicle are composed of thin-walled parenchyma cells with square, polygonal, or elongated outline. These are surrounded by epithelial cells, which are also somewhat radially enlongated. The parenchyma cells of the plumule and radicle, together with their epithelial cells, are filled with minute protein granules and fat globules. The outermost layer of the scutellum, toward the endosperm, consists of radially elongated thin-walled parenchyma cells arranged in palisade form and called epithelium. The epithelial cells of the scutellum extend to the base, to the stem, and to the coleorhiza, and are filled with protein granules and oil globules. The scutellum serves as an absorbing and conducting organ for nutrients carried from the endosperm to the embryo during germination. The epiblast, like the scutellum, is composed of thin-walled, rectangular or polygonal cells containing protein and oil particles, and is surrounded by an epithelium.
E. Starchy Endosperm
The starchy endosperm consists of thin-walled pare-nchyma cells, usually radially elongated and heavily loaded with compound starch granules and some protein bodies. The cells toward the flattened or lateral sides are either polygonal or slightly elongated (length to width ratio 0.7 to 1.4); those extended from the ventral to the dorsal side are greatly elongated (ratio 0.2 to 1.0). Generally, the radiating dimensions of these cells are shorter in long-grain cultivars than in medium- or short-grain cultivars. A central, core of small cells has isodiametric shapes ranging in size from about 45 X 50 to 80 X 105m. The length-to-width ratios of these cells in cross-section are approximately 1.0 to 1.2. These dimensions correspond to a cell area of 2,250 to 8,400 m2.
Cultivars may have 12 to 22 cells in the dorsal radius, 10 to 18 cells in the ventral radius, 10 to 17 cells in the lateral radius, and 103 to 256 cells in the longitudinal diameter. The total number of endosperm cells, however, has no proportional relation in general to the length or number of cell layers in the longitudinal diameter, except for cultivars having a very long endosperm. The number of cells is highly correlated to the size (product of longitudinal, dorsiventral, and lateral diameters) of the starchy endosperm but not to its shape (ratio of longitudinal and lateral diameters).
Endosperm cell-walls react positively to stains for protein, hemicellulose, and cellulose. The occurrence of middle lamella and two primary wall layers is suggested in some preparations. The mean thickness of endosperm cell-walls is about 0.25 m.
Cultivars differ in hardness distribution in the endosperm. Hardness distribution is represented by hardness values in kg. per mm.2 along the dorsiventral line and the lateral line crossing at the central point on the cross-section of the kernel. Hardness ratio is the ratio of hardness at the middle point of the lateral lines (halfway between the center and the periphery) to that at the central point. Indica cultivars tend to have a hardness ratio of less than l.0 Fig. 2.3. The central core is hardest,
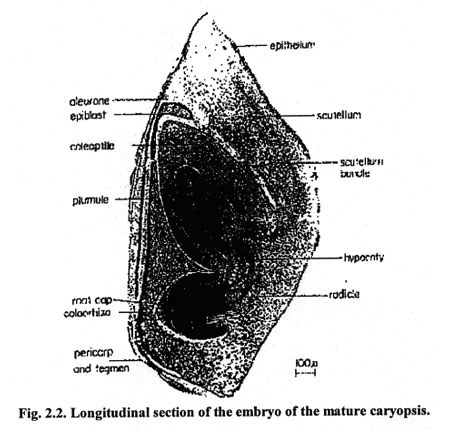
Fig. 2.3. Hardness distribution and hardness ratio of brown rice.
and hardness decreases toward the periphery along lateral and dorsiventral lines. Cells in the central core are isodiametric and arranged radially. Japonica cuitivars tend to have a hardness ratio more than 1.0. In these grains, hardness is greatest at the middle region and becomes less toward the central core and the peripheral region. In addition, the dorsiventral region is softer than the lateral region. In samples with a hardness-ratio of 1.20, the cells of the central core are uneven, markedly flattened, and disorderly arranged. A negative correlation is reported between hardness ratio and length-to-breadth ratio and also in the number of cells on the longitudinal line. The samples with a hardness ratio of more than 1.18 have a loose arrangement of cell contents at the center of the grain. Soft-grain cultivars have higher moisture contents than hard-grain cultivars. The moisture content of their grains also changes faster with a change in relative humidity.
High-protein samples tend to have a more translucent but more tan-colored grain than normal-protein samples of the same cultivar.
Rice starch granules are polygonal and compound. The granule has the structure of a pentagonal dodecahedron and ranges in size from 2 to 10 m. The polyhedral form may be due to compression of the starch granules during grain development. Waxy and nonwaxy starch have similar granule size and shape. The mean total surface area is 7,674 cm.2 per g.
Starch granules are small (2 to 4 m) in the peripheral cells of the starchy endosperm; they are fewest in the lateral and ventral peripheral cells, forming tiny clusters well separated by the surrounding dense protein material. Starch granules of the major, central portion of the kernel are larger (5 to 9 m) and occur in closely packed compound groups, several groups in each cell. Extremely small granules are found in cells near the embryo; they are usually considered devoid of starch. However, the embryo has been reported to contain minute starch granules, the largest ones being in the scutellum.
Few histological studies have been made on the rice starch granule because it is very small compared with that of corn and wheat. An electron micrograph of a hydrochloric acid-corroded rice starch granule shows the layered structure characteristic of an acid-corroded granule.
Up to 95% of endosperm protein has been observed to be in the form of discrete particles called protein bodies. These range in size from 1 to 4 m. Protein bodies are most concentrated in peripheral-lateral and peripheral-dorsal cells (whereas starch granules are fewest and least concentrated in peripheral-dorsal cells) and are found more along the periphery than in the center of each cell. Fat seems to occur in combination with protein bodies. Differences among cuitivars in their distribution in the starchy endosperm have been reported. The protein bodies are about 1m in size at peripheral cells and 2 to 3m in size in the inner cells.
Electron micrographs of isolated protein bodies indicate a layered structure such as is present in the starch granule. These alternating layers of electron-dense and electron-thin proteinaceous materials have not been completely characterized. The protein bodies have a limiting membrane and a fine granulation inside. Some of the protein bodies have a uniformly electron-dense structure which might be an unresolved form of the stratified bodies or may represent another type of protein body.
No phytate-containing bodies (globoids) have been identified in rice endosperm. Hematoxylin-staining bodies, which increase in number during grain development, were termed phosphate bodies because of their positive reaction to molybdate reagent. These are most probably protein bodies.
Opaque or chalky regions appear in the starchy endosperm of nonwaxy cultivars. When the chalky region extends to the center of the endosperm and the edge of the ventral side, this is called a white core. An opaque region occurring in the middle of the ventral side (embryo side) is called white belly or abdominal white. A long white streak on the dorsal side is called the white back.
The opacity was shown to be due to the loose packing of starch granules and protein bodies in this region. In crumbly rice the whole endosperm is practically opaque. Simple and compound granules are loosely arranged throughout the endosperm cross-section. Hematoxylin stains only a minor portion of the space between the starch granules. Hence a major portion of this area in crumbly rice is void space.
Because of the similar chemical analysis of the opaque and translucent portions of the white-belly grain the opaque portions may be structural rather than chemical in nature and probably result from collapse of the protoplasmic gel during endosperm dehydration. These opaque portions are generally softer than the translucent regions and contribute to grain breakage during milling.
Waxy rice cultivars have an opaque endosperm. Histochemical studies show that the starch granules are compound and closely arranged. Presumably the nature of endosperm opacity in the waxy endosperm is not due to the loose arrangement of starch granules. Opacity may be due to the presence in the waxy starch granules of micropores absent in nonwaxy-rice starch granules. This observation is consistent with the lower absolute density of granules of waxy starch (1.480 to 1.496) compared to nonwaxy starch (1.489 to 1.51 1) (36,1 12).
F. Milling Fractions
Abrasive milling removes the outer layers, producing milled or polished rice, and the by-products bran and polish. Bran contains more of the pericarp, tegmen, aleurone layers, and embryo than the polish, which contains relatively more starchy endosperm; and bran has a darker tan color than polish. Usually 10% by weight of brown rice is removed during milling. Bran may vary from 8.8 to 11.5% of the weight of brown rice; polish, from 1 .2 to 2.2%; and milled rice, from 86.0 to 90.0%
Bran and polish consist of fragments derived from the pericarp and tegmen together with a greater portion of the aleurone layer, part of the endosperm, and the embryo. They may be adulterated with hull fragments. Histochemical tests show that they are rich in protein, fat, starch, and free sugars. It was found that a mean bran-polish yield (eight varieties in three locations) of 9.6% from brown rice, of which 1% was endosperm and 8.6% was bran layers plus embryo.
Milled rice is slightly smaller in size than brown rice. Its outer surface is smooth, nonglistening, and waxy white. Each of the flat surfaces has two inconspicuous parallel ridges. The contents of some of the surface endosperm cells are exposed as a result of the removal of the cell-walls. The specific gravity of milled rice ranges between 1.43 and 1.47 at 30°C. and 12% moisture.
When soaked and cooked, milled rice forms transverse cracks as it absorbs water has been described changes in the grain structure on cooking. During cooking some swelling occurs and the pressure of expanding starch causes breaks in the surface of the grain. The extent and pattern of disruption depend on whether the rice is brown or milled, as well as on cultivar. In brown rice a longitudinal fissure commonly occurs along the ventral side. Other forms of kernel disruption in brown rice include splitting of the bran at irregular points around the surface, detachment of the bran, and tendency for the starchy endosperm to slough. Aleurone grains are always birefringent under polarized light in cooked rice, but never in raw rice.
In milled rice, disruption is more complete than in brown rice after a given cooking time. Longitudinal fissures always occur along the dorsal line and usually along the ventral line. The fissures can be shallow. But if they extend from the dorsal side to the core, from the ventral side to the core, or from the dorsal to the ventral side, they split or halve the grain. A simple longitudinal fissuring with a tendency to split is characteristic of long-grain cultivars, whereas splitting accompanied by crosswise segmentation or sloughing occurs in medium- and short-grain cultivars. Starch expansion and tissue splitting are exemplified in the dorsal and ventral cells where sectoring walls are far apart and protein sparse.
Longitudinal fissures in the endosperm involve the rupture of sectoring cell-walls by the expanding starch in affected cells. Halving of the grain is sometimes started by a pre-formed internal rift. Cell separation along a middle lamella is not known to occur.
Some cultivars such as Basmati 370, D25-4, and Iranian varieties elongate greatly on cooking of presoaked grain. The physicochemical nature of this unique property is not well understood. Parboiling also causes some changes in the structure of the endosperm resulting from gelatini-zation of the starch granules, disruption of protein bodies, and a harder grain texture.
G. Changes in Structure during Grain Development
After fertilization, the rice caryopsis develops much faster in the longitudinal than in the transverse axis. The caryopsis attains full length 4 days after flowering, maximum width with the 14-day grain, and maximum thickness with the 21-day sample. Grain weight increases and reaches optimum value in the 28-day sample. Tropical rices are usually harvested 30 days after flowering; in cooler climates, rices may ripen for up to 60 days. This temperature effect on the rate of grain development may explain the divergent results on morphological changes reported by various workers. The consistency of the caryopsis goes through progressive stages termed milky, dough, yellow, and mature.
The morphology of the caryopsis changes rapidly during grain development. The pericarp cells are small when the caryopsis is young but they enlarge as the grain develops later. The innermost epidermal layer, as well as the isodiametric thin-walled cells, becomes compressed and elongated. Cells above these elongated cells toward the outer epidermis fill up with chloroplasts at the dough stage. The innermost cells and those above them become tubular, probably due to compression and rapid elongation, attaining a length about 20 times their diameter. In earlier stages, two or even three layers of such tubular cells are present. But as the caryopsis matures, only the innermost cell layer persists. At maturity all the pericarp cells become greatly stretched, degenerated, and collapsed; nothing remains except their compressed walls.
The outer and inner integuments of the tegmen consist initially of two layers of cells each. The outer integument degenerates and is absent in the 5-day grain. The inner integument elongates and persists during grain develop-ment. At maturity these persistent cells of the inner integument degenerate and collapse. Varieties may differ in the character and behavior of the inner integument, as reflected in the divergent results obtained by different investigators.
The aleurone layer begins to differentiate in the dorsal area 3 days after flowering. In the 4-day grain the aleurone layer cells are nucleated. In the 7-day grain they are already fully developed. The aleurone cells are filled completely with aleurone grains and the nucleus is not always detected. Some simple starch granules may be observed in the aleurone cells of immature grain but they are absent in the mature grain. The aleurone layer is morphologicalJy complete 2 weeks after flowering.
The embryo consists of from 2 to 13 cells during the first 24 hr, after pollination. Differentiation of the coleoptile, coleorhiza, and scutellum begins by the third day. The plumule and the enclosing coleoptile are present in the 5-day grain. The vascular system of the scutellum becomes evident in the 6-day grain. Differentiation of the embryo is complete at least 13 and not more than 20 days after flowering. About 7 days after flowering, the embryo gains the capacity to germinate as a young plant.
The first division of the triple-fusion nucleus occurs 3 to 3.5 hr. after pollination, and nuclear divisions follow at a rate faster than the differentiating embryo. The endosperm has 50 to 80 nuclei by 24 hr. after pollination These form a layer at the periphery of the embryosac. Walls subsequently appear and partition the nuclei into cells. By the fourth day, the embryosac is packed with endosperm cells. Cell division is essentially complete by the tenth day and the approximate aggregate number of endosperm cells is 180,000. The dorsal side develops earlier than the ventral side of the endosperm.
Compound starch granules are first noted in the still-nucleated endosperm cells of the 4-day grain. The compound granule originates from a single amyloplast (24) rather than from several amyloplasts. Starch-granule size increases first and fastest at the center of the endosperm. The compound granules reach maximum size (39 m diameter) by 15 days after flowering. Starch granules in the exterior cell layers increase in size more slowly and are always smaller than those in the interior cells. The starch in the outermost cell layer adjacent to the aleurone layer is the most delayed; the final size of the compound granule is as small as 10m. The size of the granule increases most rapidly during the period 10 and 18 days after flowing. Individual starch granules may increase in mean granule size from 3.3 to 6.3m during grain development. The cell nucleus is difficult to detect in the endosperm cells of the mature caryopsis.
Spaces between the starch granules react positively to stains for protein, but very few protein particles can be distinguished, particularly in the center of the endosperm. Hematoxylin or bromphenol blue stains the distinct protein bodies in the 7-day grain. Hence, starch synthesis must precede protein-body synthesis in the developing rice grain. Protein accumulates from the outer to the inner layer of the endosperm, but starch accumulates in the opposite direction. Very little change in the size of protein bodies can be seen during grain development under a light microscope. Electron micrographs of ripening grain at the milky stage have revealed protein bodies 1 to 3 m in size. In the endo-sperm of the mature grain (of another cultivar), isolated protein bodies range from 1.5 to 4 m. Even at the milky stage, the protein bodies in the cells adjacent to the aleurone layer are smaller (1 m) than those found in the inner cells (2 to 3 m).
Environmental conditions can affect the rate of starch and protein deposition in the developing grain. Based on the progress of endosperm translucency, the completion of ripening is earlier in the central, apical, and ventral portions than in the peripheral, basal, and dorsal portions, respectively. A high temperature during ripening accelerates grain growth and maturation but results in a lower final grain weight of japonica rice. A high temperature (30°C.) increases the ventral radius but shortens the dorsal radius, probably as a result of a vigorous early growth and a slow later growth. Hence the ratio of dorsal-to-ventral radius is a reflection of the ripening process as well as a varietal feature. Grains from plants grown at 30°C. are also softer in the peripheral parts of dorsal and basal regions, suggesting retarded starch accumulation during the later stages of ripening. An opaque portion results from this insufficient filling of endosperm cells. The occurrence of white core is decreased by low night temperature and increased by high night temperature. These grains are somewhat bigger, especially in breadth, than normal kernels, and they also have bigger ventral radii.
Grain development is essentially complete within 3 to 4 weeks after flowering. Endosperm cracks or fissures in rough rice, some researchers claim, result from contact of the dehydrating caryopsis to liquid moisture, such as dew. Cracking results from the sudden contraction of the grain during rapid drying or the sudden expansion of the grain during grain wetting. Waxy cultivars differ in moisture content during drying when endosperm opacity is first observed.
STRUCTURE AND COMPOSITION
The Composition of typical samples of rice and rice products is shown in Table 2.4. The changes that occur as the grain is milled reflect the uneven distribution of its components relative to the distance from the surface of the kernel. As layers are successively removed, the proportion of protein, fats, and vitamins in the remaining kernel decreases, while the proportion of carbohydrates decreases. Both brown rice and milled rice are common articles of human food, rice polish and rice bran are predominantly used as animal feeds.
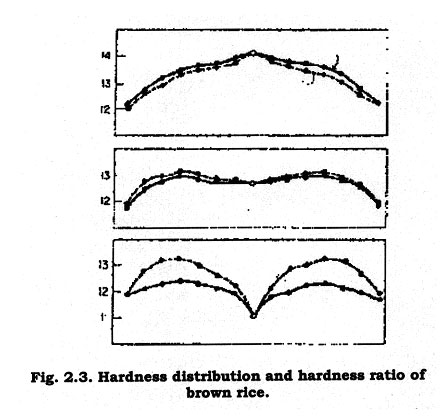
Structure of the Rice Kernel
As harvested, rough rice consists of the outer covering tissues comprising the hull, or husk, and the inner kernel, i.e., the caryopsis, or brown rice. The caryopsis is enveloped by the caryopsis coat consisting of the pericarp, seed coat, and nucellar layers. The fibrous pericarp consists of compressed cells and is about ten microns thick. Just inside it is the seed coat, a layer of cells having a thick cuticle and representing what remains of the inner integument. Proceding inwardly, the next layer, only about 2.5 microns thick, consists of the remains of the nucellus; it has a thick cuticle adjacent to the seed coat cuticle. Inside the nucellus layer is the aleurone, which completely encloses the endosperm and the embryo. It is composed of 1 to 7 layers of rectangular parenchyma cells and varies in thickness in different parts of the caryopsis. The structure of a typical rice kernel is shown in the drawing reproduced here as Fig. 2.4. Bran Institute).
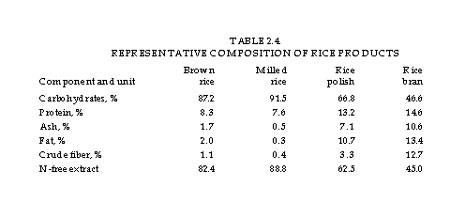
Fig. 2.4. Cross-section of a grain of rough rice.
The aleurone cells that surround the endosperm are filled with protein-rich aleurone grains with a single membrane containing globoids or phytate bodies in a protein-carbohydrate matrix, and lipid droplets or spherosomes around a nucleus that is centrally located. Aleurone cells that surround the embryo are modified, lacking aleurone grains, and having fewer spherosomes than other aleurone cells. They also contain filament bundles.
The embryo or germ is relatively small and is located on the ventral side of the caryopsis. Within the germ are embryonic leaves and an embryÂonic primary root joined together by a very short stem. The embryo is bound to the endosperm by the scutellum (cotyledon). Immature cells of scutellum and mesocotyl are found between differentiated parenchyma cells.
The endosperm, which in the mature caryopsis is much larger than all the other tissues combined, consists of thin-walled, radially-elongated parenchyma cells, which are packed with compound starch granules and protein bodies. Protein bodies, which are covered by single membranes, are more abundant in the outer cell layers where the compound starch granules are small. The compound starch granules are in double-membraned amyloplasts. The husk constitutes perhaps 18 to 28% of rough rice, while the remaining 72 to 82% is brown rice. The weight distribution of the brown rice grain components is pericarp 1 to 2%, nucellus and seed coat 4 to 6%, embryo 2 to 3%, and endosperm 89 to 94%. About 6 to 10% of brown rice is removed in abrasive milling, the by-products being bran and polish.
Important Components
Proteins.- The nutritional quality of rice protein is high relative to that of most other cereal proteins. This is due to rice's relatively high content of lysina, which is the first limiting amino acid. Most of the so-called storage proteins in rice kernels are found in discrete protein-rich bodies (aleurins) in the endosperm, similar to the situation in many other seeds. This protein is largely insoluble in water. Most of it- 80% or more in milled rice- is the type called glutelin, soluble only in dilute acids or alkalies. The second most abundant type of protein is the salt-soluble globulin. Both globulins and glutelins are composed of an unknown number of molecular species which are generally isoelectric around pH 7.
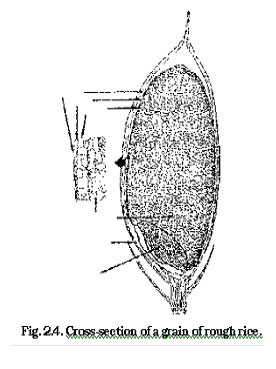
The amount of protein and the amino acid composition of the protein of rice will vary with growing conditions and variety. It has been found that the same variety of rice grown at different locations will vary considerably in amino acid composition. As the percentage of nitrogen increases in the grain, due to growing conditions for example, there is a tendency for the percentage of lysine in the protein to decrease. Different rice varieties grown at the same location will also contain proteins varying in amino acid composition. Furthermore, the distribution of the different amino acids is not uniform within the grain. Milling not only causes a decrease in the total protein of the kernel, but also a change in the amino acid pattern of the remaining protein. Generally, the decrease of lysine on milling is disproportionately large, while there is a relative increase of isoleucine, leucine and valine.
Table 2.5 gives values for ranges in the amino acid composition of brown rice, milled rice, rice bran, and rice polish, as found by one investigator (adapted from Juliano 1980).
Starch.-Milled rice will contain from about 84 to over 90% starch (dry basis). This component is present in the form of angular granules measuring about 2 to 7 microns in their largest dimension. Many of these particles are roughly pentagonal in outline. In size and shape, they are somewhat like the granules of oat starch, but differ in that few if any rounded particles occur. The hilum is centric and indistinct, and birefringence is weak.
In the seed, starch exists in compound granules containing up to about 150 of the smaller particles. These clusters are firmly bound together by a water-insoluble protein matrix which can be dispersed, and the granules released, by various alkaline steeping methods.
The viscosity behavior is similar to that of corn starch. Nonwaxy starch from indica rice contains 21 to 33% amylose, while that from japonica rice will contain a lower amount (17 to 19%) of the linear fraction.
There is a wide variation in iodine affinity and viscometric molecular weight depending on the variety from which the amylose is taken.
Rice starch preparations contain small amounts of lipid. Fats determined by acid hydrolysis amount to about 0.7 to 0.8%. A large part of this material consists of fatty acids which cannot be removed by petroleum ether but dissolve readily in methanol or 80% dioxane.
Waxy (glutinous) rice has been grown in Asia for many centuries. In some countries, the major portion of the crop consists of these varieties. The principal differences between waxy rice and normal rice depend upon the starch,-that from waxy rice consisting of almost 100% amylopectin and thus staining red with iodine solution, while non-waxy starch contains a substantial amount of amylose, and stains blue with iodine. Typically, waxy rice will" contain 2% or less of amylose. Both the flour and the purified starch from waxy rice has been found to have advantages for certain food and indus-trial uses. Gravies, sauces, and puddings based on this starch are very resistant to syneresis during freezing and thawing.
Lipids.-There is very little lipid material in milled rice, perhaps 0.3 to 0.4% by acid hydrolysis could be considered average. Rice bran contains a much higher percentage of lipids, perhaps 21%. Nearly all the studies of rice lipids have concentrated on rice bran oil, and there is a subs-tantial trade in the fatty materials extracted from rice bran and refined.
The glycerides of rice bran oil contain mostly fatty acids having even numbers of carbon atoms from 14 to 20 as well as the odd-numbered fatty acids of chain length 11, 13, and 15. The odd-numbered chains were present in the small amounts of 0.2, 0.6, and 0.9%, respectively, in one sample. Gas-liquid chromotography indicates that bran lipids have significantly higher mean contents of linoleic and linolenic acids, but-lower contents of myristic, palmitic, palmitoleic, and stearic acids, than the lipids of milled rice. The iodine values of bran lipids were reported to be significantly higher than for milled rice lipids, and the lipids of indica varieties had lower iodine values than did this component in japonica rice.
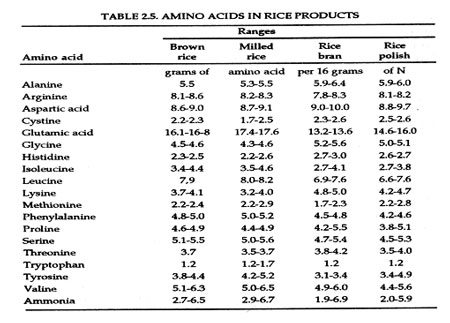
Vitamins.-So far as vitamins are concerned, rice is an important source of only thiamin and niacin. The following data are mg per 100 g, first figure for raw, second for parboiled rice. Thiamin was present at levels of 0.13 and 0.15, niacin at levels of 1.54 and 3.2, pyridoxine at a level of 0.14 for raw, and riboflavin at levels of 0.04 and 0.044. "When compared on an energy basis with the RDA for adult women and when rice is a large part of the diet (2000 Kcal), brown rice would give more than sufficient niacin, thiamin, and phosphorus... Protein needs would be nearly enough- 91%. . .but calcium and riboflavin needs would be low, both about 23% [zinc about 60%]. Milled rice, however, would be deficient in all nutrients with only protein, niacin, and phosphorus supplying more than 50% of the RDA." A more complete survey of reported values of vitamins in rice and rice components is given in Table 2.7. This table is with only the medians of the ranges being taken from the original data.
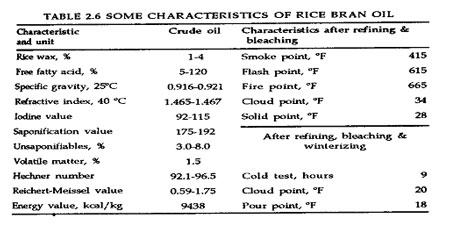
*Values calculated as mg per kg, 14% moisture basis, except * micrograms per kg.
Minerals.-Milled rice is not an important source of minerals by any reasonable standard. The content of ash in a large series of samples ranged from 0.26 to 1.95%, dry basis, with a mean of about 0.65%. There is a considerably higher concentration of minerals in the bran, but the availability of at least some of these is adversely affected by the high concentration of phytin in the bran. Table 2.8 summarizes the mineral and phytate content of rice kernels.
Calcium, iron, potassium, sodium, silicon, magnesium, sulfur phosphorus, and minor amounts of other elements have been found in rice-ash. Potassium and phosphorus are the most abundant mineral elements in rice. Represen-tative figures would be 88 mg and 140 per 100 g dry weight respectively. Appreciable amounts of silicon are found in the mature rice plant, and slight amounts in milled rice; the function of this element in the plant and its value, if any, in human nutrition is unknown.

Sodium, magnesium, and calcium were found at mean concentrations of 8.1, 28, and 25 mg, respectively, in the whole kernels of six varieties of milled rice.
Of the trace minerals, iron is present in brown rice and white rice at 1.8 and 0.9 mg per 100 g, dry weight basis, respectively, and zinc is present at 1.6 and 1.4 mg per 100 g, as is basis, respectively.
Other Constituents.-Sugars, hemicellulose, nucleic acids, pigments, phytin, and numerous other substances have been found in small amounts in rice. Phytin, the principle phosphorus compound in rice is said to constitute more than 8% of the bran in some samples.
There is a small, but important, amount of sugar in rice. Brown rice will contain about 0.8 to 1.4% total sugars. Reducing sugars are typically near 0.1%. Parboiled rice can be expected to contain around 0.7 to 1.1% total sugars, and about 0.16% reducing sugars. Milled rice will typically contain much lower amounts of total sugars, about 0.4%, and perhaps 0.06% reducing sugars.
The amount of fiber found in rice varies greatly, depending on the method of analysis, the variety of rice and its growth conditions, the processing which has been applied to the rice, and other factors. Among the reported values for fiber in brown, milled, and parboiled rices are 0.9, 0.3, and 0.2%, respectively.
A concise summary of nutrients and other components to be found in a typical sample of long grain brown rice is included in Table 2.9.
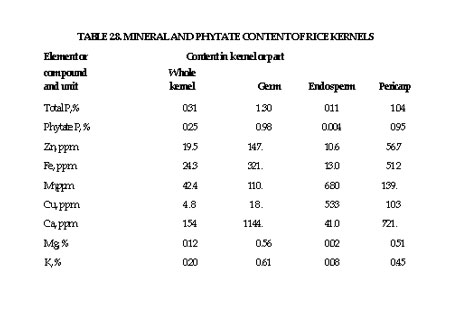
1Where no values are stated for a component, or component has been omitted from the listing, there are inadequate data available.
CRITERIA OF RICE QUALITY
I. INTRODUCTION
Rice quality, like that of numerous other food products usually evaluated according to its suitability for a specific end-use for a particular consumer. The quality of rice is closely related to the quality of its milled whole kernels, since essentially all of the domestic rice crop is milled to a high degree. Virtually all rice, in one or more of its many prepared forms, is used as food for human consumption. Some of the most important uses and processing applications of rice include boiled or steamed rice (milled raw rice, milled parboiled rice, brown rice, and quick-cooking rice) for consumer use, prepared in the home, institution, or restaurant; dry breakfast and baby-food cereals; brewers' rice, used as a carbohydrate source in the manufacturing of beer; canned rice, such as in soups, puddings, and mixes; quick-cooking and other convenience and specialty food products; rice flour, as a thickener in sauces, gravies, and puddings; rice starch, for industrial and other processes; and preparations for certain types of fermented foods. Because of its many diverse applications, rice that is satisfactory for one use may not be suitable for other uses. Quality testing, therefore, is primarily a matter of determining whether the rice is suitable for a particular use and whether it meets specific requirements of cleanliness and purity.
Some standards for rice quality are observed in all countries, although in some the requirements are simply that the grain be clean and dry. In a few countries, legislative measures have been taken to prescribe well-defined grades but, despite numerous attempts at establishing uniform standards, there is still no generally accepted basis for trading rice throughout much of the rice-growing world. The most international trade is conducted on the basis of quality as determined generally by visual examination. In most of the local markets of the world there are no fixed scales of allowances for impurities and other defects. A general increase in trade between countries has emphasized the need for establishing universal grades and standards, but little progress has been made.
Consumer acceptance and preference, with respect to eating, cooking, and processing qualities, are important in judging rice, since they vary from country to country and from region to region consumers prefer rice grain that cooks dry and fluffy, with kernels that retain their conformation and remain separate after cooking. Others prefer rice that cooks moist and chewy, with the grains tending to cling together. Since different cultural groups prefer various cooked textures, there is a demand for all types in domestic and foreign trade. Processors of rice also require different textural qualities for use in various kinds of prepared rice and rice-containing convenience-food products. In some processes the dry and fluffy-cooking types are preferred, while in other products, the moist and chewy-cooking types are required. In other countries, the preference range is from waxy or glutinous to a high degree of fluffiness.
Milling yields are based on the amount of whole milled kernels (usually the milled product of greatest value) and all sizes of broken kernels obtainable from a unit of rough rice. Many factors influence milling yield; and the rice processor, usually the miller, must also judge the quality of rice for milling on the basis of variety, moisture content, the presence of red rice, peckiness and other kernel damage,- foreign seeds and other material, chalky kernels, and mustiness or other undesirable odors.
Wide inherent plant differences existing in rice quality are due in part to natural evolution. In more recent years, specific differences have appeared as a result of planned breeding. Marked differences in rice quality also exist owing to environmental and cultural practices during growth. In some instances, differences due to these factors are greater than inherent factors.
Factors influencing rice quality which are independent of inherent, cultural, and environmental differences include those associated with handling, storage, and the incidence of foreign material. For example, rice that has been stored for long periods, or stored under unfavorable conditions for shorter periods, may result in lower yields of processed products and contain varying degrees of objectionable odors and flavors which would decrease its suitability for human consumption. However, a short period of maturation after harvest, under proper storage conditions, is considered desirable by most users to allow important changes in enzy-matic activity to occur.
Thus, rice quality is considered to have two general meanings: 1) milling, cooking, and processing qualities, which refer to suitability of the grain for a particular end-use; and 2) physical quality, which means cleanliness, soundness, and freedom from foreign material. Usually physical quality and milling, cooking, and processing qualities are interrelated in that certain market grades of rice are more suitable than others for consumer products.
The nutritional quality of rice is, of course, of para-mount importance and concerns all aspects of rice quality. However, it is not the purpose of this chapter to present it here.
The scope of this chapter is: 1) to define the more important criteria used in evaluating rice milling, cooking, and processing qualities; 2) to explain how the criteria are effectively used in determining and predicting quality; 3) to describe some of the procedures and instruments used in such determinations; and 4) to present the criteria used in determining the grade of a unit of rice.
II. OBJECTIVE VERSUS SUBJECTIVE MEASUREMENTS OF CRITERIA
An ever-increasing trade, domestically and between markets of the world, emphasizes the need for uniformly accurate procedures for judging rice quality. Unless the procedures are objective, the criteria of quality that can be accurately and uniformly measured and interpreted are difficult to describe. Although many objective methods are available for evaluating rice quality, some important criteria must still be determined subjectively. For several factors, such as odor, flavor, taste, and texture (mouth appeal), no practical objective method or instrument exists. Other factors, such as color, can be measured objectively, but the instruments required are expensive or do not have the ability to adjust for other factors, such as damaged kernels, which influence color. The human eye has the capability of making this adjustment; however, because of the vast differences in sensitivity of individual eyes and human errors in judgment, reproducibility of results is very poor. The authors of this chapter have attempted to cite objective methods, or instruments for making objective measurements, whenever these are practical or appropriate. Progress is being made in developing new tests and instruments, but much more research is needed. Therefore, rice buyers, processors, inspectors, and researchers currently must rely on subjective measurements of several quality factors.
III. VARIETIES
Rice varieties (Oryza saliva L.) are divided by grain size and shape into three types, known as short-, medium-, and long-grain rice. Historically, and now through planned breeding, each grain type is associated with specific milling, cooking, and processing characteristics. Raw milled rice of typical long-grain varieties usually cook dry and fluffy when boiled or steamed, and are generally preferred for use in prepared products, such as parboiled rice, quick-cooking rice, canned rice., canned soups, dry soup mixes, frozen dishes, and other convenience-type rice-containing foods. Typical short- and medium-grain types, on the other hand, cook moist and chewy, and are preferred for manufacturing into such products as dry breakfast cereals or baby foods, and for brewing uses. Therefore, since domestic and world trade associates long-, medium-, and short-grain varieties with specific milling, cooking, and processing characteristics, it is essential for new varieties of each grain type to have the same (or improved) qualities as the varieties they replace.
There are a number of rice varieties of each grain type in commercial production, and new ones are continually in the process of being developed and released. Previously, in rice-breeding programs, most emphasis was placed on grain yield, grain type and appearance, milling yield, plant type, nitrogen responsiveness, and resistance to diseases and insects. Now, particular attention is being paid to the cooking and processing qualities of rice for specific uses.
With but few exceptions, the grains of all rice varieties in commercial production are described as translucent, non-scented, nonwaxy (common) types which contain varying ratios of amylose and amylopectin starch, and are considered by the trade as being bland in taste. One exception is a limited production of waxy (glutinous) rice. This rice, also called "sweet rice", is characterized by an opaque 'endosperm containing virtually all amylopectin-type starch. Waxy rice is produced primarily for specialty products and for certain ethnic-group uses. Another exception is the scented (aromatic) rice produced on small acreages as a specialty product. This rice gives off a popcorn-like aroma during cooking, and has a flavorful and appetizing taste similar to the much-sought-after "Basmati" class of varieties in India. Its grain is also described as translucent and contains both amylose- and amylopectin-type starch.
IV. GRAIN SIZE, SHAPE, WEIGHT, AND UNIFORMITY
The physical dimensions of rice kernels are of vital interest to those engaged in the many facets of the rice industry. These dimensions are important in marketing and grading, in developing new rice varieties, in cleaning and grading equipment, in drying operations, and in processing.
Rice is marketed under three market types, designated as long-grain, medium-grain, and short-grain. Varieties of each grain type must conform within rather narrow limits to the size and shape specifications established for that type. Thus, grain size and shape are among the first criteria of rice quality that breeders consider in developing new varieties for release in commercial production. If the variety does not conform to recognized standards for grain size, shape, weight, and uniformity, it is simply not considered for release. In early developmental stages, close visual examination of the grain is made to ascertain that its configuration conforms to that of other commercially acceptable varieties of the same grain type. Inherent grain defects, such as irreguilarly shaped grains, sharp pointed extremities, oversized germs, and deep creases which cause bran streaks in milled rice, must also be eliminated in early developmental work, as these defects reduce the milling yields of rice and detract from the general appearance of the processed product.
While grain type can be visually classified, more exact measurements are needed for classification and for critical comparison of varieties. Rice grains may be objectively classified into grain-type categories based upon three physical quantities: length, shape, and weight. Length is simply a measure of the rough-, brown-, or milled-rice kernel in its greatest dimension. The shape is determined by a ratio of two of the three dimensions - length, width, and thickness. Probably the most meaningful of these is the length:width ratio, since it is used in sizing rice with slotted sieves or so-called precision graders. Finally, the grain weight (size) is determined by taking the mean of the weight of a 1,000-kernel representative sample. Uniformity of grain size and shape is calculated by determining the coefficient of variation for each dimension of a representative sample.
Some attempt has been made to subdivide the types into finer gradations, such as long-slender and medium-slender. However, these distinctions may not be justified, since there is considerable overlap between long and long-slender and between medium and medium-slender types.
The weight of rice kernels can vary considerably with moisture content, type of soil where grown, fertilizer treatment, and weather conditions. Consequently, a better or complementary measurement may be that of density. A device known as an air comparison pycnometer, which permits rapid density measurements of granular materials, is now commercially available.
V. COLOR AND TRANSLUCENCE
The color is used as a criterion of quality for all forms of rice. The evaluation, however, is generally performed on whole-kernel rice after it has been well milled. Colors of raw milled rice range from white to dark gray or rosy. Rosy color occurs when rice is milled with a large quantity of red rice. The bran from the red rice stains the endosperm of the cultivated variety. Parboiled rice is usually .graded from "parboiled light" to "parboiled dark", depending upon the degree of coloration resulting from the parboiling treatment. The trend in commercial processing is toward "parboiled light", which is accomplished through various parboiling techniques and bleaching processes.
Color of raw milled rice is often referred to as general appearance. The latter term is probably more descriptive, since it takes into account not only the hue, lightness, and saturation, but also various defects which contribute to the tristimulus factors of color. For example, smut may contribute toward a distinct gray tint, whereas heat damage may contribute toward a particular hue. General appearance, as a quality factor, can be determined only by a visual examination, since it is composed of many different factors which the human eye can integrate into one hypothetical, but nevertheless useful, value.
Color may also be an indication for other quality criteria. For example, the International Rice Research Institute showed that high-protein rice was less white (more tan-colored) when cooked than rice with normal protein content. In the quality-evaluation studies color ("L" value for lightness) "was negatively correlated with surface lipids-in all grain lengths-and positively correlated with water uptake and sediment." However, the study also recognizes that surface lipid content and color are partly dependent upon the degree of milling, which may also affect the water absorption of rice.
Several attempts have been made to use optical instruments for measuring the color and degree of milling of rice. It is shown that optical-transmission measurements are generally unsatisfactory for color measurements. Reflectance measurements, however, are quite reliable for measuring color. A color and color-difference meter equipped with a large-angle viewing head, such as the Hunterlab Model D25, is probably one of the most suitable commercially available instruments. The tristimulus color factor known as "lightness" (Hunter L) correlated highest (r=0.946) with visual color as determined by official rice inspectors visual deter-minations. Other simple reflectance meters, are now commercially available. The use of one or more of these instruments would probably satisfy the needs of an objective method of measuring the color of rice.
Another quality factor closely related to color is that of translucence. Nonwaxy (common) varieties of rice have a vitreous, translucent endosperm of varying degrees of apparent hardness. This trait is highly desired by the rice industry, and rice breeders pay particular attention to developing new varieties having bright, clear, highly translucent milled kernels free of inherent chalky spots. In the vitreous types, chalky grains also occur when rice is harvested too early and some kernels are not mature, and when the rice is grown under environmental and cultural conditions conducive to chalk formation. Translucency in rice is usually subjectively determined by close visual examination of the well-milled kernel. In contrast to the vitreous translucent types, another kind of rice known as glutinous, sweet, or waxy rice is characterized by a completely opaque endosperm.
VI. TEST WEIGHT
Closely related to the criterion of kernel weight is another factor called the test weight per unit volume. It is directly related to the bulk-density of the grain. Test weight is useful as an index to milling outturn, in measuring the relative amount of dockage or foreign material in a given lot of rice, in measuring the amount of shriveled or immature kernels, and in estimating the weight contained in a storage bin of given volume. In countries using the metric system, the test weight is expressed in terms of kilograms per hectoliter. Multiplication factors for converting test weight from any one of the commonly used systems to any other are given below.
I
From | To | Multiply by Factor |
b./Winchester bu. |
Ib./Imperial bu. |
1.032 |
Ib./Imperial bu. |
Ib./Winchester. bu. |
0.969 |
Ib./Winchester bu. |
Kg./hectoliter. |
1.287 |
Kg./hectoliter. |
Ib./Winchester.bu. |
0.777 |
Ib./Imperial bu. |
Kg./hectoliter |
1.247 |
Kg./hectoliter |
Ib./Imperial bu. |
0.802 |
VII. MOISTURE CONTENT
Moisture content has a marked influence on several facets of rice quality. Its effect is of primary importance on the keeping properties of rice during storage, because under practical storage conditions moisture level is usually the factor most responsible for controlling the rate of deterioration of the grain. Only a few days are required for wet rice to deteriorate badly, whereas dry rice can be kept for relatively long periods when properly stored. Moisture contents commonly accepted for "safe" storage of rough rice are 13% for less than 6 months, and 12% for long-term storage.
Milling quality is greatly influenced by moisture content of the rice at harvest time, during drying and storage, and while milling to prevent excessive breakage. To command premiums, rice must be of high milling quality, and for this high quality it must be harvested at the correct stage of maturity, and properly dried, stored, and milled under moisture conditions optimum for minimum breakage.
It show that how moisture content of grain can be of direct economic importance based on the amount of water contained in a carload of wheat of 14% moisture versus 8% moisture content. This relation could apply to rice as well as other grain; but because of the precautions taken in producing, harvesting, and artificially drying domestic rice, fluctuations in moisture content are not usually as great as for some other grains.
Other quality factors, associated with rice milling, cooking, and processing characteristics, may be adversely affected by excessive moisture. During storage these characters usually change more rapidly in high- than in low-moisture rice. Also, uniformity of grain moisture content (individual kernels), as well as limitations on moisture level, is a primary consideration for rice to be used in dry breakfast cereals, parboiling, and other processed rice and rice-containing foods.
Several methods are available for determining the moisture content of rice. The basic method used is 130ºC. 1 hr. air oven method. Another basic method used frequently is the vacuum-oven method for cereals. The principal disadvantage of these conventional methods is the relatively long time required to run the test. Where rapid results are necessary- such as in driers, mills, and for grading purposes-the electric moisture meters are widely used and, under controlled conditions, are sufficiently accurate for most control work. For the most satisfactory results, these instruments must be calibrated with one or more of the conventional methods
VIII. IMPURITIES AND DAMAGED RICE
The quantity and character of impurities and damaged kernels in rice obviously affect the value of a lot. In addition to reducing the quantity of usable rice, lowering milling yields, and affecting the value of rice for food, certain impurities can also damage the processing equipment. For example, stones can severely damage rubber-roll shellers.
A. Dockage
Dockage is defined as all matter other than rice which can be removed readily from the rough rice by the use of appropriate sieves and cleaning devices. This includes underdeveloped and shriveled kernels as well as small pieces of rough rice which are removed in proper separation of the dockage and which cannot be recovered by proper rescreening or recleaning. Other impurities which may be difficult to remove because they have approximately the same size, shape, and density as rice are classified as objectionable materials. Certain weed seeds, and metal and glass fragments fall into this category.
Generally, brown and milled rice are sufficiently clean to make the dockage test unwarranted.
B. Damaged Kernels
Damaged kernels are defined as kernels and pieces of kernels of rice which are distinctly discolored or damaged by water, insects, heat, or any other means. When parboiled-rice kernels are found in nonparboiled rice, they are classified as damaged rice. Whole kernels of parboiled rice found in nonparboiled rice, which are as dark as, or darker in color than, the interpretive line samples for heat-damaged kernels are classified as heat-damaged rice. This distinction is made because heat-damaged rice is considered to be a more serious defect than some other forms of damage.
Damage to rice can occur in the field prior to harves-ting, during drying operations, or during subsequent storage and handling. Wet weather, after the rice has matured, can cause various types of staining, and promote the growth of fungi. Excessive heating from either artificial drying or high-moisture conditions in storage can result in the development of red to brown stains known as heat-damaged kernels. Certain types of storage fungi can also produce this type of discoloration. Damage may also result from insect infestations, which can seriously reduce the quality of rice for human consumption. Finally, mechanical damage can result in lowering the yield of both total and whole-kernel milled rice, because of breakage of the endosperm or weakening of the kernel by fissures.
The only reliable method presently available for determining the amount of damaged kernels in rice is visual inspection. The rice inspector must be trained to recognize various types of damage that may occur in rice and he must be able to judge in each instance whether the damage is sufficient to be considered under the applicable standards. This often involves hand picking, separation, and counting. Electronic seed counters are now available to assist in the counting operation, but the entire process remains slow and tedious.
Various color sorters have been manufactured to separate particles of a distinctively different hue from particles of another hue. However, these devices are not satisfactory for grading for two reasons: 1) since all commercial sorters use reflected light, they fail to detect particles only slightly different in hue or particles that are opaque, and 2) all commercial color sorters are production-type machines which sacrifice accuracy for high volume. However, research and grading laboratories must now rely on visual examination to detect and evaluate damaged kernels in rice.
Some of the commercial electronic color sorters are in use in rice mills, primarily for separating peck (discolored) kernels from normal kernels in parboiled rice. Parboilers of rice usually consider any distinctly discolored kernel, regardless of source of discoloration, as pecky rice. Peck in parboiled rice is a severe problem, since the parboiling process tends to intensify color development in the damaged kernels and most of these kernels must be removed before use. Some parboilers set their own requirements as to the amount of peck which can be tolerated in rice purchased for parboiling. Usually this tolerance is very low (1.5% and less) because of the difficulty and expense incurred in removing pecky kernels from the parboiled product.
C. Chalky Grains
Chalky grains, as mentioned previously, occur under unfavorable environmental and cultural conditions and when rice is harvested too early and includes immature kernels. In some varieties the panicles do not mature uniformly, so that some kernels are immature while others are overripe; in certain varieties there is a genetic tendency for chalkiness in all kernels. Chalky rice not only detracts from general appearance, but is usually very weak and, therefore, breaks up easily during milling, with a subsequent reduction in the yield of milled rice. An exception to this occurs when rice -is parboiled, since the gelatinization process tends to strengthen the kernels. However, excessive chalkiness, even in rice to be parboiled, may not be desirable for modern parboiling processes because of the possibility of product nonuniformity as a result of overprocessing some kernels while underprocessing others. Other manufactured rice products, such as quick -cooking rice and dry breakfast cereals, may be similarly affected by excessive chalkiness. The processors usually place limitations on the amount of chalk permissible in rice purchased for manufacturing these products.
The Grading Service classifies chalky kernels as kernels which are one-half or more chalky. The type of chalk (location of chalky spots on the endosperm), as well as the amount of chalk, is important to some processors of rice, as certain types of chalk affect the quality of their particular processed product more than other types. Depending on the location of chalk on the kernel, the chalky spots may be referred to as "white belly", "white core", and "white back". Determination of the amount and type of chalk in rice is currently made by visual examination.
D. Red Rice
Red rice (Oryza saliva L.) is a serious weed pest in many rice-growing areas. It resembles cultivated varieties but tillers more profusely and shatters easily after maturity. The grains show a marked dormancy following ripening and have the ability to retain their viability in the soil for several years.
The red seed coat (pericarp), usually in the form of a firmly adhering bran, detracts from the appearance and market value of the commercial product. The bran of red rice adheres to the endosperm so firmly that it is difficult to remove in milling without causing excessive breakage of the cultivated variety.
As with damaged and chalky kernels, red rice must currently be determined by visual inspection. Color sorters are efficient in separating red rice (with a substantial amount of bran adhering) from white rice, but are rather inefficient in separating kernels of red rice having only light streaks of the bran remaining.
E. Seeds or Kernels
Seeds or kernels, either whole or broken, of any plant other than rice are classified as seeds. The rice industry usually considers as objectionable those kinds of seeds difficult to remove by screening, sieving, or aspiration because of their similarity to rice in size, shape, and density. Examples of objectionable seeds are from the senna family, Sorghum almum Parodi and Aeschynomene virginica (L.) B.S.P. Seeds of this type must be removed by hand separation.
F. Odours
Odours in rice other than those normally associated with clean, sound, rough, brown, milled, and parboiled rice of naturally scented and nonscented varieties are considered objectionable and usually drastically lower the value of the rice. Included in the same category with musty or sour odours are moldy ground odours, insect odours, and rancid and sharp acrid odours. Commercially objectionable foreign odours for grading purposes include those of fertilizers, hides, oil products, skunk, smoke, and decaying animal and vegetable matter. Odours of fumigants, except under circumstances specified are also considered as objectionable foreign odours. Determinations of odours are made subjectively by trained inspectors on the rice as a whole, usually at the time of sampling.
IX. MILLING QUALITY
The criteria of rice quality described above and the subjective and objective methods discussed in determining them are of value primarily because they are indicative, to some extent, of the milling quality of rice and of the suitability of rice for specific cooking and processing uses as human food. However, other more definitive criteria are required to evaluate rice milling, cooking, and processing qualities, and these are described in subsequent sections of this chapter.
A. Milling Yield
Milling yield is one of the most important criteria of rice quality. The objective in milling rice is to remove the bran layers and germ with a minimum of breakage of the whole kernels, with the preservation of most of each kernel in its approximate original shape. It therefore becomes very important from a marketing standpoint to determine the milling yield of rice accurately.
In determining the milling yield of rough rice, foreign material (dockage) is first separated. Then the rice is shelled to remove the hulls, then milled to remove most of the bran layers and germs, and finally sized to determine the yield of whole and broken kernels. Two values of milling yield are commonly used in grading: whole-kernel rice yield and total milling yield. The whole-kernel yield is the quantity of whole kernels of milled rice (usually well-milled) that can be obtained from a given quantity of uncleaned rice. Total milling yield is the quantity of whole and broken kernels of milled rice that can be obtained from a given quantity of uncleaned rough rice.
As commercial milling machinery is gradually improved, a problem arises in using any one device to determine accurately the milling yield of rice. Most commercial rice-milling facilities, therefore, rely upon methods and laboratory mills adapted to fit their individual needs. However, for official grading, a standardized procedure is necessary. A machine which would give the ultimate maximum milling yield would probably best serve the needs of the rice industry and trade.
A large number of factors can affect both total and whole-kernel milling yields. Some of these-such as foreign-material content, chalky- and immature-kernel content and damaged-kernel content-are obvious. Other factors which can seriously affect the milling yield are variety, grain type, growing conditions, harvesting method (including combine efficiency), control of drying operations, and-of particular importance-effects due to adsorption and desorption. For some time it has been known that rice can be easily damaged by conditions occurring during rapid drying or in rewetting of the kernels through moisture adsorption. It is shown that rapid changes in environmental conditions can cause rice to crack or fissure milling yields are affected by environmental conditions which exist in a rice mill. Therefore, the environmental conditions in a rice-grading laboratory probably should be controlled. Furthermore, the condition of the rice sample should perhaps be controlled with respect to moisture content and temperature prior to milling. Neither of these procedures is commonly practiced in grading laboratories at the present time, except that samples of rice to be tested are usually allowed to equilibrate for a few hours with laboratory conditions prior to milling. Most of the rice-inspection laboratories also allow hot milled rice to temper or cool slowly in semi-closed containers prior to separating whole kernels from broken kernels.
The final step in determining an estimated milling yield is the separation of whole-kernel milled rice from broken kernels in the sample: A high quantity of brokens is indicative of poor milling quality, whereas a high percentage of whole kernels is indicative of high quality. All rice markets discriminate greatly against broken rice.
Various types of sieves, indent cylinders, and graders have been developed for separating the various sizes of brokens.
B. Degree of Milling
Degree of milling is a measure of the extent to which the bran layers and germ have been removed from the rice endosperm. A majority of the consumers prefer well-milled rice with little or no bran adhering to the endosperm. Ironically in countries where rice is the principal food, the preference is generally for well-milled rice, which is decreased in nutrient value since the proteins, fats, vitamins, and minerals are concentrated in the germ and outer layer of the starchy endosperm. Furthermore, in rice that is overmilled, there is a reduction in both the total and whole-kernel milling yields, with a subsequent loss in market value.
In general, there are four degrees of milling: well-milled, reasonably well-milled, lightly-milled, and under-milled. These descriptions are nebulous in that there is no precise definition for them.
Determinations of the degree of milling are made largely by visual inspection both in commercial milling operations and in grading laboratories. A technician can be trained to become quite efficient in a particular system, but from a world-market viewpoint an objective method is needed.
A number of objective methods have been proposed. Some of these consist of dye-staining techniques designed to emphasize the residual bran for easier visual observation.
Probably one of the most reliable objective methods presently available is a chemical procedure. It was found that the amount of fat extracted from whole milled rice was linearly related to the amount of bran removed up to about 6% of the original rice by weight. While this method is generally reliable, it requires considerable chemical skill and a suitable, fat-extraction apparatus, and is rather time-consuming. Therefore, this method has received only limited use in rice-milling facilities and grading laboratories. It has received wider use as a research method because of its accuracy.
In the early 1950's, several investigators developed optical methods for measuring degree of milling of rice. In general, the instruments were of the reflectance type and measured degree of whiteness or lightness. In many cases, whiteness can be affected by factors other than the amount of bran remaining on the rice a very low degree of correlation was found between any of the tristimulus color factors and degree of milling. However, a marked change in absorbance associated with degree of milling exists at 660 and 850 nm. A technique and an instrument known as the Rice Ratiospect, which measures the ratio of the transmittance at these two wavelengths, has been developed by Stermer and co-workers. Extensive tests have shown that instrument readings are highly correlated with surface lipids (r = 0.837). Some difficulties are being experienced 'mouth appeal' of cooked rice. It remains for someone to develop an instrument or method for measuring the combined qualities of cohesiveness, chewiness and smoothness, which are the important elements in sensory evaluation of a bland cooked product such as rice." Basic research on these and other factors is essential for developing rice varieties of improved quality. Also, as new uses of rice are developed and as new or improved techniques in rice processing become available, some of the quality characteristics now needed may change or require modification. Consequently, rice cooking and processing qualities require constant evaluation.
C. Physicochemical Tests
Following is a brief discussion of some of the more important physicochemical test methods routinely used as criteria of rice cooking and processing quality. The starch components of rice, particularly the amylose content or amylose: amylopectin ratio, its temperature of gelatini-zation, and its pasting characteristics, are largely responsi-ble for major differences in rice cooking and processing behaviors. The influence of protein in rice cooking and processing behaviors is generally unknown. As mentioned previously, high-protein rice tends to have a creamier appearance and it was shown that high protein content increased the cooking time while lowering the water-absorption capacity. The protein content of rice, in conjunction with many other factors, appears to influence the parboil-canning stability of rice. In general, the higher the protein content the greater the canned-kernel stability. The lipid components of rice are important factors influencing storageability of rice, and in this regard are discussed in this monograph in other chapters on raw and processed products. Some processors have low specifications for fat content as a precaution against the development of rancidity, and for the high viscosity effect it has in certain brewing applications.
The amylose content in rice is considered the single most important characteristic used in describing and predicting rice cooking and processing qualities, and numerous reports point out this relation. The investigation first showed that the preferred long-grain domestic varieties had the highest amylose contents, whereas the short- and medium-grain types had somewhat lower amylose contents. Glutinous or waxy varieties contain virtually no amylose.
Amylose content in rice may be empirically estimated by the starch-iodine-blue test. However, it has been shown to be of limited use for certain types of rice.
The gelatinization temperature of rice is also related to rice cooking and processing qualities. Amylograph studies have shown that typical short-and medium-grain varieties gelatinized at lower temperatures than did the typical long-grain types. These results were confirmed by granule swelling and by BEPT determinations. Gelatinization temperature of rice can be determined by the amylographic procedure and by BEPT determination. It is most often estimated with the alkali spreading-value test, which permits classification of rice varieties into high, intermediate, or low-gelatinizing types. Low-gelatinizing types are preferred in boby foods, specific brewing uses, and many dry breakfast cereals. Intermediate-gelatinzing types are characteristic of typical cooking long-grain varieties. High gelatinizing types are generally considered undersirable for most cooking and processing uses.
The pasting quality of rice, as determined with the amylograph showed appreciable differences among varieties of known cooking and processing behaviors. Typical long-grain varieties with the highest amylose contents usually showed the highest increase in viscosity of the cooled paste at 50ºC. This character is of particular value in identifying those varieties of superior parboil-canning characteristics and quick-cooking properties. Typical short and medium-grain arieties generally exhibit somewhat lower cooled paste viscosities at 50ºC.
Varieties with kernels suitable for parboiling are also desired in home-cooked rice and are utilized widely in manufacturing quick-cooking and other rice products. Thus, determining the parboil-canning stability of rice provides a means of evaluating its use for many products. Canned-kernel stability is evaluated subjectively according to clarity of broth, kernel splitting, and fraying of edges and ends.
Another important quality criterion of rice to be parboiled is that of hull color. Rice varieties U.S. have either light (straw) or dark (gold) colored hulls. Light-colored hulls are preferred by parboilers of rice because they do not impart as much color to the processed product as do dark-colored hulls under similar parboiling conditions. Light parboiled rice is also preferred by most consumers. In rice-breeding programs, hull color is now a prime consideration in developing new varieties which go into the parboiling trade-an example of how industry requirements can cause changes to be made in the quality criteria of rice.
RICE DRYING
I. INTRODUCTION
Rice ordinarily is harvested at moisture contents above safe storage levels, so additional drying usually is needed. The grain is harvested together with its husk or hull (lemma and palea) and is called rough rice or paddy. It is dried in this form since husked rice cracks badly when stressed by the usual drying procedures, and since rough rice stores better than does brown rice. Husk removal is a preliminary step in rice milling.
Unbroken rice kernels are preferred for many uses and their market value is greater than that of broken kernels. This makes it highly desirable to minimize any kind of stress that may result in kernel breakage. Because the outside layers dry faster than the inside, internal stresses develop when a rice kernel is drying. These stresses may become great enough to cause checks or cracks if moisture is removed too quickly, and checked kernels are likely to be broken when the rice is milled. Checking due to rapid drying may occur in the field before rice is harvested.
A. Harvesting Methods
Direct combining is the harvesting method used for nearly all rice produced. The major farm machinery manufacturers design and build combines especially for use in rice fields. In general, these are self-propelled, high-powered machine's with headers and with large tires for operation under extremely muddy conditions. Rice combining is a highly mechanized operation which includes cutting heads from stalks, separating grain from straw, removing chaff from grain, and elevating the threshed rice to the grain tank. The grain tank is emptied periodically into a field cart and the cart delivers the rice to a truck. The rice is transported by truck to a drying facility. Artificial drying of threshed rice is a supplemental operation, made necessary by combine harvesting.
In countries where combines are not used, the plants are cut with a variety of machines or hand tools. The cut stalks may either be threshed immediately or after some degree of drying has occurred in a windrow or shock.
B. Optimum Harvest Time
The time of harvesting affects the quality and quantity of rice yield, regardless of the drying, storage, or milling method used. Although the optimum time depends primarily on climatic conditions, it also depends on variety characteristics, field conditions, and the possibility of losses owing to rodent, bird, insect, or microbiological activity. Criteria used to determine the proper harvest time include maturity in relation to panicle color, age of the paddy crop, and moisture content of the paddy. None of these criteria is completely reliable and considerable judgment is required to balance all the critical factors involved. A number of studies have been made to determine the best time to harvest.
Total milled rice continued to increase as rice was harvested at progressively lower moisture contents to, 12% although not rapidly beyond 25%. Chalkiness decreased with harvest moisture content but did not drop below 1% until the moisture content at harvest was below 26.2%.
It was found in a study that highest head yields occurred when harvested at a moisture content between 24 and 16%. A small variation was noted in the optimum range of moisture contents among each of the varieties, and variation also was noted within a variety for early, medium, or late planting. In general, the optimum harvest moisture content was slightly higher for early-planted rice compared with late-planted rice. Total yield, bushel weight, and germination showed a tendency to increase when rice was harvested at progressively lower levels of moisture content.
C. Preharvest Chemical Drying
Chemical desiccants have been applied to rice as a preharvest treatment to speed drying of rice in the field. In research, mixtures containing sodium chlorate and magnesium chlorate were applied to rice as sprays when the moisture content of the kernels was between 20 and 27%. This treatment was effective in reducing the moisture contents of kernels as much as 1% per day, depending on weather conditions, especially relative humidity. It was found that application of desiccants reduced the milling yield, but the germination was unaffected. Interest in this practice has dwindled because of unfavorable results.
II. RICE-DRYING TERMINOLOGY AND FUNDAMENTALS
The rice industry uses a number of specialized terms and measuring units. Some of those associated with drying are discussed and their specific use in the context of this chapter explained.
A. Kinds of Rice
Rough rice is the harvested product with hulls intact. In some foreign countries it is called paddy. Brown rice is the unmilled product, but with hulls removed. Milled rice is the product from which the bran layer and a part of the germ have been removed. Head rice is whole kernels of milled rice. Mention of rice in the following sections of this chapter should be understood to be a reference to rough rice. Other kinds will be specifically designated.
B. Milling Yields
Milling yields are expressed in pounds or on a percentage basis. The number of pounds of unbroken grain milled from a specified quantity of rice is the head yield, and the number of pounds of all milled rice (broken plus unbroken) is the total yield. The number of pounds of head yield and total yield per cwt. of rough rice are the same numbers as those for expressing milling yield on a percentage basis.
C. Weights
Rough rice weights are expressed in bags or cwt (100 lb., 45.36 kg.), barrels (1 bbl., 162 lb., 73.46 kg.), and metric tons (2,200 lb., 1,000 kg.). Unless otherwise stated, moisture content is not a factor in these terms, but they are usually corrected to 1 2 to 14% moisture when used to express acre yields of paddy to be milled.
1 metric ton = 1 ,000 kg. = 2,200 lb. = 22 bags = 48.89 bu. = 13.38 bbl.
Test weight is a measure of the bulk density of rough rice.
D. Moisture Content
Moisture content is commonly expressed on the wet basis, i.e., the percentage of water present in the wet grain. It is determined by the air-oven method, by distillation, or by means of meters which relate electrical resistance or dielectric properties of the grain to its moisture content.
The air-oven method requires that grain, previously ground to pass a 20-mesh screen, be dried at 130°C. for 1 hr. The distillation method, called the Brown-Duvel test, measures the volume of water in milliliters condensed in a graduated cylinder after 100g. of grain and 150 ml. of mineral oil are heated to 200° C. in a distillation flask. The Tag-Heppenstall meter measures the electrical resistance of grain as it is crushed between two corrugated rolls. The Motomco moisture meter is a dielectric type in which this property is determined for a sample placed in a circular cell. The last three methods can be carried out by semi-skilled personnel and are widely used at commercial rice driers. The Brown-Duvel test takes 30 to 40 min.; the Tag-Heppenstall test, about 20 sec.; and the Motomco test, about 1 min.
It is less common but equally correct to express moisture content as a percentage of the dry weight of a material. This expression is preferable for analytical purposes, because the weight of dry matter remains constant, but the combined weight of dry matter and moisture continually changes as drying proceeds. References to moisture content (m.c.) in the remainder of this chapter are on the wet basis (w.b.) unless specifically designated as dry basis (d.b.). Conversion equations for these two expressions are:
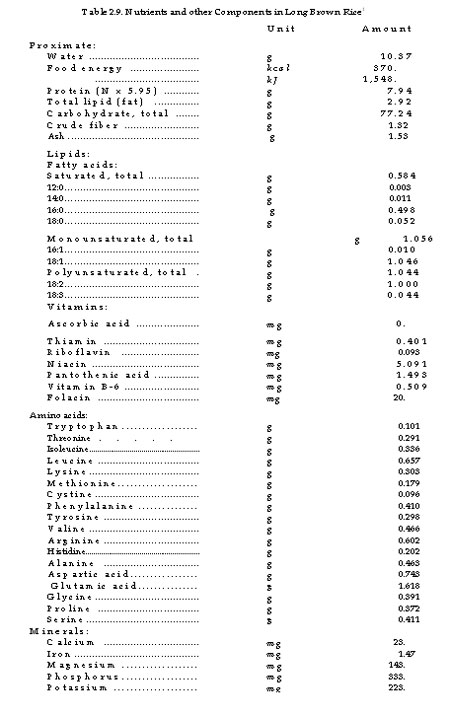
E. Equilibrium Moisture Content
Like other grains, rice is hygroscopic, and will gain or lose moisture until it is in equilibrium with the air it contacts. The equilibrium moisture content is dependent primarily on the relative humidity, but it varies to a lesser degree with air temperature. Rice losing moisture through exposure to air at any given temperature and relative humidity has a slightly higher equilibrium moisture content than does rice adsorbing moisture by exposure to the same air. Values of equilibrium moisture content are different for rough rice, brown rice, milled rice, and bran.
F. Drying-Rate Computation
A great many studies have attempted to develop formulae for computing grain drying rates, but none has been universally accepted. While work is continuing on this phase of rice-drying research, empirical methods are presently used in design computations. The drying rate is dependent upon the interrelationships among the following parameters:
- Air temperature and relative humidity (or any other two physical or thermal properties of moist air)
- Airflow rate
- Rice moisture content
- Rice temperature
- Moisture distribution within a rice kernel
- Rice grain type and variety characteristics
- Bed depth of rice in drier
- Resident time of rice in drier
When any two physical or thermal properties of air are known, a point on a psychrometric chart is fixed and other properties are established. These include wet- and dry-bulb temperatures, humid volume, relative humidity, absolute humidity, vapor pressure, and enthalpy. Further discussion of air properties is beyond the scope of this book, but they are discussed at length in many standard texts.
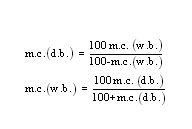
Airflow rate for drying is an expression of the volume of air per unit of time in relation to a quantity of grain. Common expressions for rice are cubic feet per minute (c.f.m.) per cwt. (or bushel or barrel), and cubic meters per minute per metric ton.
1 c.f.m. / cwt. = 0.624 m.3/min./metric ton
Rice temperature is an important consideration in drying-rate computations when a considerable part of the energy, for moisture evaporation is supplied by a reduction of rice temperature.
Moisture distribution in a rice kernel affects the drying rate because of a time lag in the movement of moisture from the interior to the outer surface. The drying rate is faster at the beginning of a drying operation when the surface of a kernel is moist than it is after the surface moisture has been reduced. Differences in the resistance to the internal movement of moisture owing to variations in size and shape may partially explain why the grain-type and variety characteristics of rice affect the drying rate.
The bed depth is the thickness or depth of rice through which drying air passes. Bed depth affects the drier holding capacity, drying rate, and uniformity of drying. Fast, uniform drying and small holding capacity are associated with a shallow bed depth; slow, uneven drying and large holding capacity are associated with a deep bed.
The resident time or time of exposure to drying air is the time required for a kernel to travel from the top to the bottom of a columnar drier. Resident time is inversely proportional to the rice flow rate or throughput rate. It is adjusted by changing feedroll speed in some driers or the rocker-arm frequency in others. The drying rate tends to increase as resident time is reduced.
G. Drying Methods
Air is the medium commonly used for absorbing moisture from rice. Both natural convection and forced airflow are used. The former is found most widely on a worldwide basis, but it is not compatible with highly mechanized harvesting methods.
Forced-air driers may use either unheated (natural) air or heated air. They may be designed for either batch or continuous-flow operations. Some driers are designed to utilize a deep bed of rice, whereas others use a shallow bed. In some driers infrared energy, rather than air, is used to heat rice. Dielectric heating of rice has been used in other driers for the same purpose.
III. FORCED-AIR DRYING
Rice produced is dried in different kinds of forced-air driers. DeepÂbed driers, using unheated or moderately heated air, commonly utilize depths of 2.4 to 3.7 m, but occasionally greater depths are used. In continuous-flow, heated-air driers, the thickness of the rice pack is (0.l 5 to 0.30 m.). Depths of (0.46 to 0.61 m.) are often used in batch driers.
In deep-bed driers, dangerous moisture gradients within individual kernels are minimized because moisture is removed slowly. In heated-air, continuous-flow driers, a multipass procedure is used to avoid excessive drying stress. During each pass, rice is exposed to heated air for a short time and only a small amount of moisture is removed, mostly from the outer portion of the kernel. The moisture within a kernel is equalized while rice is stored in a bin following a drier pass. This is known as tempering. The multipass procedure is efficient because the drier is used only when the kernel surfaces are relatively moist; tempering bins are used for the slow movement of moisture to the surface.
A. Deep-Bed Driers
Deep-bed drying is widely used as an on-farm method and as an off-farm finish-drying step. Bin driers are designed for both drying and storing without any need for moving the grain during these operations. They differ from other storage structures in that provisions are made for a fan and an air-distribution system. Bins built of steel, wood, or concrete have been adapted for drying. The main consideration is to provide a tight structure in order to prevent leakage of air and moisture through bin floors and walls.
Air distribution systems commonly used are a perforated floor (sometimes called "false floor") or different arrangements of ducts and laterals. The perforated floor provides the most even distribution of air, but each of the systems provides adequate air distribution for drying.
Both axial-flow and centrifugal fans are suitable for bin drying. The vaneaxial fan consists of a fan wheel within a cylinder fitted with air guides or vanes, either ahead of or behind the wheel. It is designed to move air over a wide range of volumes and pressures. The tubeaxial fan is similarly constructed, except that the vanes are omitted. It will move a wide range of air volumes, but at moderate pressures.
Centrifugal fans are differentiated by the blade design on the revolving wheel. Fans with forward-curve blades are generally of lighter construction and less expensive than the other two kinds. Straight-blade fans are suitable for operating against a high static pressure because of their rugged construction. Both forward-curve and straight-blade fans are subject to overloading with decreasing system resistance. A fan with backward-curved blades has a self-limiting horsepower characteristic. This makes it more suitable than the other two types for use in deep-bed drying where a wide range of static pressures may be encountered because of varying rice depths.
Airflow direction in bin driers is usually upward and the last rice to dry is at the top where it is readily accessible for sampling and visual examination.
Drying tests made under favorable weather conditions indicated a satisfactory airflow rate was 7.2 c.f.m. per bbl. (4.4 c.f.m. per cwt., 2.8 m.3 per min. per metric ton) for rice at 20% m.c. However, to provide a factor of safety for unfavorable weather and wetter rice, the recommended airflow rate was 3.5 m.3 per min. per metric ton). The wettest layer should be reduced to 16% m.c. within 15 days to prevent mold activity if the rice temperature is in the range between 65°. (18° to 24°C.). The risk of mold damage is greatly reduced when rice moisture content is below 16%. The following fan-operating schedule was recommended: Start the fan as soon as the air-distribution system is completely covered and operate it continuously until the moisture content of the top foot, after completion of filling, is reduced to 16%. After this, operate the fan only when the relative humidity is less than 75%, and continue drying until the top foot is reduced to 12.5%.
In the area, where the climate is drier and nights are cooler, drying tests indicated that satisfactory airflow rates were 1.5 m3 per min. per metric ton at 20% m.c., and (2.5 m3 per min. per metric ton) at 25% m.c. It was recommended that the fan be operated continuously except during heavy rains and dense ground fog until the moisture was reduced to 15% or less or until the third week of October, after which the fan should be operated only when the sun is shining or light conditions are bright.
The drying time with unheated air varies greatly with weather conditions. In two drying tests, both with 8-ft. rice depths and airflow rates of 3.5 m3 per min. per metric ton, 19 days were required to dry the top foot to 12.5% m.c. during favorable drying weather; but 40 days were required when much of the weather-was unfavorable for drying.
The estimate of air delivery in a bin drier may be made by measuring the static pressure under the perforated floor with a manometer and noting the grain depth. Table II shows the air-delivery rate for different static pressures and depths of rice. For other types of installations, a traverse over fan inlets with an anemometer will give the average air velocity, which, when multiplied by the inlet area, will give total quantity of air delivered.
B. Supplemental Heat
Supplemental heat may be used to improve the effectiveness of deep-bed drying during prolonged periods of high humidity or cold weather. However, it may result in overdrying and quality loss, with the additional
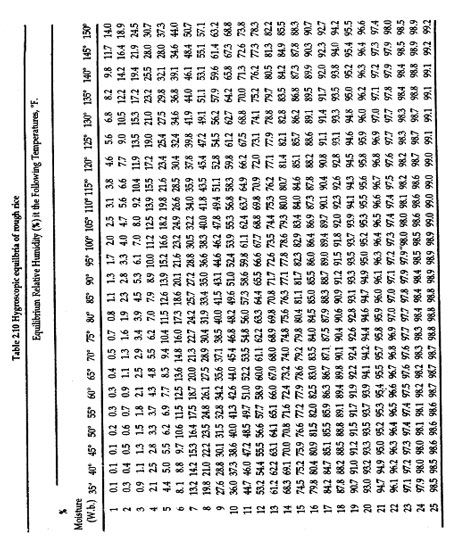
disadvantages of greater equipment costs and the need for closer management. Both natural and LP gas are used as fuels. Combustion products are mixed with the drying air. Gas burners and safety controls are dependable and inexpensive.
C. Materials-Handling for Bin Driers
Mechanical unloading of a round-bin drier is accomplished with a sweep auger discharging through an opening in the center of the perforated floor to a conveyor under the floor. Rice which will not flow by gravity is brought to the center opening by the sweep auger. The screw conveyor installed below the perforated floor moves rice from the bin. A variety of unloading equipment is available for different bin systems.
A common arrangement for several drying bins is to group them around a large circle with a cone-shaped dump pit in the center. The bins are filled by a portable auger loader which moves grain from the pit and discharges into any of the bins or into a truck. The bins are unloaded through a center opening and rice is conveyed to the dump pit for further handling using the portable auger loader.
Another arrangement is to install the bins in a straight line together with two horizontal conveyors, a dump pit, and a bucket elevator. Rice is moved from the dump pit with the bucket elevator, which discharges into a horizontal conveyor or a truck. This conveyor is located above the bin roofs and it spouts into any of the bins. The other conveyor, near ground level, moves rice from any of the bins to the dump pit. A variation of this method is to provide a bucket elevator tall enough to spout directly into all of the bins and eliminate the top horizontal conveyor.
Spreader, are used to eliminate the manual labor required to level rice in bins. Some spreaders sling grain at high velocities and may increase packing. There is a tendency for heavy material to be slung to the outside and light material to settle in the center. Nonuniform airflow and drying may result if slingers are not operated carefully.
Vertical stirring augers mounted in a track, which move through a bin and mix the grain in any location at frequent intervals, are available under various trade names. They loosen grain which may have packed excessively during loading, and provide a mixing action which results in a more uniform moisture content over the entire depth. With adequate mixing, a greater amount of supplemental heat can be safely applied to speed drying. Research is needed to further evaluate the beneficial effects of stirring augers for rice drying.
D. Continuous-Flow, Heated-Air Driers
Most of the rice crop is dried at commercial drying installations or rice mills in continuous-flow, heated-air driers by the multipass method. The products of combustion are commonly mixed with the drying air. These driers are of the columnar type, in which rice flows by gravity from the top to the bottom of the drier, where it is discharged. The rate of discharge is mechanically regulated by feed rolls or oscillating rockers. When the rice follows a straight path the drier is classified as a nonmixing type; when it is diverted from a straight path, it is classified as a mixing type.
The nonmixing type is simple in design Fig. 2.5. Rice flows between two parallel screens, usually set 6 to 9 in. (0.15 to 0.23m) apart. Heated air flows horizontally through the screens and rice. Since the grain cannot be blown out, comparatively high velocity air can be used. The grain tends to flow straight downward, so that the layer closest to the screen through which the air enters is always exposed to hotter, drier air than the layer near the exhaust side. The effect is similar to drying in a static bed with a bed-depth equal to the distance between the screens. However, mixing does occur as the rice is discharged and conveyed to tempering and storage bins.
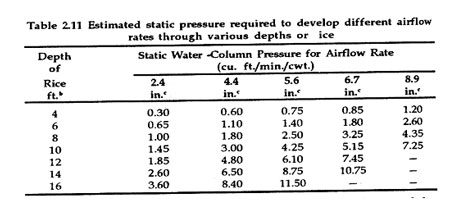
Mixing-type driers are of many designs. One common type, consists of a vertical compartment across which rows of air channels are installed. The air channel cross-section is often an inverted "V". One end of each channel is open and the other is closed. Alternate rows are open to the hot air; intervening rows are open to the exhaust-air plenum chamber. Alternate rows are also offset, so that the channel tops split the grain stream as it descends, causing some mixing action. Generally, all rows run in the same direction, but in one design alternate rows are at right angles to each other. This also results in mixing and provides rigid construction.
Another mixing drier is the baffle type. The grain stream flows between shaped sheet-metal baffles, which create turbulence and mixing.
In mixing-type driers, chaff and light material are blown into the exhaust air stream. If the air velocity is excessive, some grain may also be blown out, and driers should be designed to recover sound kernels that are displaced. However, the removal of chaff and other light material improves- rice quality. As the grain descends, individual kernels encounter successively hotter and cooler air, and no kernel is continuously exposed to the hottest air.
The nonmixing-type drier characteristically uses a higher air velocity and lower air temperature than the mixing type. Typical airflow rates are l 12 to 262 m.3 per min. per ton in the nonmixing type, and 44 to 97 m.3 per min. per ton in the mixing type. Air temperatures up to 130°F. (54°C.) are commonly used in nonmixing driers, whereas temperatures up to 150°F. (66°C.) are used in the mixing-type driers.
In a typical multipass operation in either kind of drier, rice is exposed to heated air for 15 to 30 min. for each pass and moisture content is reduced by 2 to 3% of the dry weight. Between passes the rice is moved from the drier to a bin tempering for 4 to 24 hr. The total elapsed time for multipass drying generally exceeds 24 hr. and may be as long as only 4 to 5 days, but the grain is in the drier only 1 to 2 hr.
E. Tempering
The tempering period following a drier pass provides time for moisture to move from the interior of a rice kernel to the exterior, where it is available for evaporation. California tempering tests showed that rice temperature affected the time required for satisfactory moisture equilibration. Rice cooled to 75ºF (24°C.) immediately after drying required 6 hr. for adequate tempering, but only 4 hr. were required for tempering rice at 105°F. (41°C.). An observation showed a continuous gain in drying efficiency for tempering up to 24 hr. The gain was very rapid during the first 6 hr., but only minor after 12 hr.
Some driers are equipped with an ambient-air cooling section directly below the heated-air section. However, when rice emerges from the heated-air section, the hull may be dry enough to reabsorb moisture from the cooling air. For this reason, cooling rice before or during a tempering period is not a recommended practice when this period is of short duration. If the next drier pass must be delayed for a considerable length of time, the rice should be cooled as much as possible to inhibit molding, souring, and yellowing. In this case, cooling by aeration while the rice is stored in a bin is recommended. Tempering periods are extended deliberately by some drier operators to take advantage of the additional drying that accompanies slow cooling by aeration.
F. Combination System of Drying
Almost all California driers have coupled heated-air multipass drying with deep-bed ambient-air drying for at least part of their paddy. The grain is dried to 16 to 18% moisture by the multipass method and then finish-dried with ambient air while in storage. This system has good potentialities for minimum rice breakage and large drying capacity because: a) drying is faster and rice breakage lower when heated-air drying is used only at high moistures, and b) breakage is less when ambient-air drying is used for further drying. The combination system provides an inexpensive way to expand plant capacity, since the deep-bed driers provide low-cost storage facilities as well as additional drying capacity. California finish-driers hold as much as 4,500 metric tons, with bed depths as high as 30 to 35 ft. (9.1 to 10.6 m.). Some drier operators have encountered difficulties when paddy was improperly cleaned or leveled, when airflow was too low, when paddy was too wet, when air humidities were too high for an extended period, or when chaff was allowed to segregate during filling. In general, however, results have been good.
G. Batch Driers
Batch driers which dry in one pass are widely used for corn and some small grains. For rice, one-pass drying is excessively slow because low air temperature must be used to avoid kernel checking.
A batch drier with a capacity of 5 metric tons of rice was tested. The rice was loaded between two concentric perforated cylinders, 5 and 8 ft. (1.5 and 2.4 m.) in diameter. Heated air at a rate of 6,500 c.f.m. (183 m.3 per min.) was-blown into the inner cylinder, passed through the 18-in. (0.46-m.) thickness of rice, and emerged from the outer cylinder. A vertical auger in the center of the drier continually moved rice from the bottom to the top. The mixing which resulted kept rice at a uniform temperature and moisture content. Drying time varied from 6 hr. with 120°F. (49°C.) air to 3 hr. with 150°F. (66°C.) air. Rice dried with 120°F. air had a milling yield equal to that of rice dried with unheated air, but the head yield was reduced by 8% when 150°F. air was used.
H. Other Drying Methods
Fluidized-bed drying uses an air velocity high enough so that rice is suspended, totally surrounded, and sometimes transported by the drying air. This method has been used in laboratory-scale drying tests, but has not been used commer-cially.
Laboratory rice-drying experiments showed that heating rice by infrared radiation resulted in rapid drying without excessive breakage. Kernels spread in a single layer were dried at rates ranging from 0.49 to 3.60% m.c. per min exposure to infrared. Head yield varied directly with the total time rice was exposed to infrared radiation and with the maximum temperature to which it was heated. Head yield varied inversely with the radiation and with the maximum temperature to which it was heated. Head yield varied inversely with the radiation intensity and with increasing rates of moisture removal. Germination decreased as temperature and rate of moisture removal increased. In more recent research, infrared radiation has been used for rapidly preheating rice before a pass through a heated air drier. This method for increasing drier capacity is discussed later in the chapter.
Dielectric energy provides another means of heating rice. In laboratory scale drying rests, rice was dried from 21 to 13.5% m.c. in three passes, each of 5 min. duration. The advantage of this method are uniform application of heat in the rice mass and rapid drying. This method has not been used for large-scale drying because of excessive equipment costs.
IV. COMMERCIAL RICE DRYING
A. Types of Enterprise
A grower has a number of choices for drying or marketing his rice. He may: a) send it to a custom-drying facility which will dry the rice for a fee, b) send it to a cooperative drying organization of which he is a member, c) sell the freshly harvest (green) rice to a mill, or d) dry it in a farm drier.
In general, custom driers, cooperatives, and mills use the heated-air multipass drying method. On-farm driers may be deep-bed, batch, or continuous-flow.
Cooperatives prorate the cost of running the plant among the members and may act as sales agent for the growers. After the rice is sold, the growers are compensated for the dry weight of grain they delivered. The price is adjusted for milling yields, grade, and dockage.
Some mills, buy a grower's rice before it is harvested. The grower then harvests and delivers undried rice to the mill for drying. Payment generally is made on the basis of green weight with an upper limit of moisture content at which the mill will accept delivery.
B. Receiving and storing undried Rice
Handling operations involved in receiving rough rice at commercial driers from farm trucks were studied. These operations included weighing, sampling, dumping, and conveying to storage bins.
The samples from trucks are used only for moisture-content determinations when the identity of the lot is preserved throughout the drying operation. When lots are commingled, the milling yield and other quality factors as well as moisture content are determined from these samples. Some operators estimate grade and milling yield from green samples. Others use a more elaborate system in which green samples are dried in a sample drier and then graded at government laboratories or by substantially official government grading methods. A "shrink chart", which shows the shrinkage in weight owing to moisture removal, is used for the computations. The shrink chart may include handling losses in addition to moisture losses. Dockage losses are computed separately.
Straw, weed seeds, unfilled heads (blanks), and other extraneous materials provide focal points for heat damage and interfere with operation of conveyors and driers. Many drier operators remove large foreign materials such as straw and joints either before green rice goes to the holding bins or while on its way for the first drying pass. Cleaners equipped with rotating or vibrating screens and aspirators frequently are installed above columnar driers so that smaller foreign material is removed during each drier pass.
Rice harvested at high moisture content and high temperature is subject to quality damage when drying is delayed. The presence of foreign material, frequently wetter than the rice, increases this danger. Drying should be started as soon as possible, preferably within 24 hr. after harvesting. However, this is not always possible, especially when the receiving rate at the peak of the harvest season exceeds drying capacity. Whenever green rice must be held in bulk storage, aeration should be used to dissipate heat from respiration and microbiological activity and to maintain the rice at ambient temperature. The rice moisture content, ambient temperature and airflow rate are factors which affect the predicted safe storage time.
C. Method for Increasing Drier-Facility Capacity
The rice crop matures during a relatively short period. This leads to heavy demands on drying plants. Wheather conditions have have a pronounced effect on the operating method selected. In damp or rainy weather the crop usually is harvested at a high moisture content and is subject to rapid spoilage. The drier is then called upon to dry the paddy in as short a time as possible and to remove more than the normal moisture while operating under the handicap of high humidity air. In hot and windy weather the rice may dry too rapidly in the field and deteriorate because of checking. Effort to avoid weather damage result in heavy deliveries to the drying plant.
Fluctuations in rice acreage from year to year pose another problem. Drying facilities take time to build are costly, and may be difficult to finance. For these reasons, it is generally preferable to utilize existing facilities to their maximum capacity rather than add to them in order to satisfy a temporary demand for increased drying capacity. Methods for increasing drier-facility capacity, while at the same time maintaining quality, include: a) more passes or increased throughput rate (or both) at higher air temperatures, b) increased airflow rate, c) supplementary drying during the tempering period, d) infrared preheating, d) changes in handling methods, and f) use of a combination of heated-air drying followed by deep-bed finish-drying.
Laboratory-scale experiments were conducted to develop a basic pattern for multipass rice drying and to provide infor-mation in a form to be used regardless of the type of driers in the plant. These tests included determinations of the effects of drying-air temperature and humidity, airflow rate, and number of drying passes on milling yields and drying time. The tests were performed in 2-and 6-in. deep static beds with controlled-humidity air.
In general, it was concluded that: a) when air temperature is increased without changing the number of passes, drying is faster but head yields are lower; b) when the number of passes is increased without changing air temperature, drying is faster and head yields are higher; c) when a proper combination of high air temperature and number of passes is chosen, high head yield and fast drying result. It was further concluded that no single set of drying recommendations will fit all driers because of design, climatic, and varietal differences. Therefore, each drier operator must determine the best drying conditions for his driers. Two of the following variables are required to define optimum drying conditions for any particular drier: heated air temperature, discharge air temperature, feed or discharge rate of rice (or resident time in the drier), number of drying passes, temperature of dis-charged rice, and amount of moisture removed per pass. A test procedure for determining optimum drying conditions was published. Later, a statistical analysis of the magnitude of the errors which occur in these test procedures was published and procedural revisions for decreasing the errors were suggested (43) and tested.
The practice of supplementary drying by aeration of binned rice following a drier pass has been widely adapted at commercial driers. Rice is cooled by aeration while stored in a bin following each pass through the drier. As it cools, rice generally gives up moisture, even when cooling air is at high relative humidity. The amount of drying is a function of both the amount of cooling (difference between rice temperature and ambient-air temperature) and the moisture content of the rice before it is cooled. The average amount of drying was more than 1% (d.b.) per cooling period for rice-drying tests spanning a 10 year period.
Each cooling period should be long enough so that rice temperature at all locations in a bin approaches the ambient-air temperature. A study of cooling times at different airflow rates resulted in a curve for predicting cooling time, the equation of which is:
Cooling time, hr. = K/airflow rate
The value of K was determined to be approximately 15.4 with airflow rate expressed as c.f.m. per cwt., rice moisture content above 14%, and rice temperature 20º to 35°F. higher than ambient-air temperature.
By the preheat method, rice is heated rapidly while falling between gas-fired infrared heaters on its way to a continuous-flow drier. With preheating to 140°F. and retention time in a continuous-flow drier of 2 to 3 min. with a drying air temperature of 120°F., rice moisture content was reduced approximately 2% in each pass, with no apparent damage to milling yield. Total resident time for drying from 20 to 12% m.c. is estimated to be four 3-min. passes, or a total of 12 min. This compares with resident times of 15 to 30 min. per pass and total resident times of 60 to 120 min. to do the same amount of drying by conventional methods.
Studies at commercial driers showed that drying capacity can be increased by improved handling methods. Every drying facility has three basic operations: receiving, drying, and shipping. In a well-planned facility, all three can take place simultaneously. In some poorly planned facilities the flow of rice to one or more drying unit, must be stopped at times in order to ship or receive. The drying capacity of such a facility can be increased by the installation of additional conveyors so that all operations can be carried out simultaneously. A significant loss in productive drying time results when emptying a drier of one lot and load-ing it with another lot. One method of reducing this nonproductive time is to load drying units from large capacity garner bins. As a drying unit is emptying, a garner bin is filled with rice from the next lot to be run. After a unit is empty, a slide gate is opened and the drier refilled within 1 to 2 min.
Nonproductive time for emptying and filling drying units is greatly reduced by commingling lots produced by different growers. This method is widely used where a large percentage of drying is done by cooperatives. Segregation of lots is by variety and moisture content. However, commingling is possible only if growers will accept the drier-management's determination of delivered weights, moisture contents, milling yields, and other quality factors as a basis for adjusting payments.
Methods and equipment used for drying rice in other highly industrialized countries are similar. However, most of the world's rice is grown and consumed in areas where fields or paddies are small and mechanical drying is primitive or nonexistent. In these areas the general practice is to cut the mature rice plants by hand and sun dry the paddy either before or after threshing. This applies whether the crop is grown in flooded paddies or on dry fields.
A. Sun and Shade Drying
Each country or area has its own method of sun drying, depending on the peculiarities of customs, climatic conditions, varieties, and labour supply. The simplest and most common method is to lay the cut plants in the field, either in swaths or loose bundles, until the paddy is dry. A frequent variation is to gather up the partially dried plants after a few days and arrange them in shocks, stooks, or heaps for a second drying. The second drying may also be done on drying floors or mats after threshing. Another method is to thresh immediately after cutting and dry the threshed grain.
In drying unthreshed grain, the farmer may try to protect the grain from suncracking by arranging the cut plants in rows with the panicles covered by the straw of another row, or shocks may be built of upright bundles with a capping bundle to deflect rain and provide some shading.
The cut plants are often piled in large stacks-and suffer from lack of air movement. This leads to sprouting, discoloration, and microbiological damage.
Racks of varying degrees of complexity sometimes are used for hanging unthreshed plants that have been tied in small bundles. Designs vary from two simple pole tripods with a pole resting between them to more elaborate arrangements of poles in tiers. Most racks are designed to permit air movement through the drying plants.
Small quantities of threshed wet paddy may be spread on woven mats or left in winnowing baskets for further drying. Large lots are spread on drying floors of different types which vary with the locality. Floors may be made of beaten earth which are sometimes covered with lime mortar or dung, or they may be made of bricks or concrete. Drying takes a long time on beaten-earth floors and paddy is likely to become soiled. Concrete floors crack and the rough surface causes a certain amount of paddy breakage during handling. The temperature of both concrete and brick floors may be high, causing additional damage.
Layers of grain vary in depth according to the moisture content, air temperature, quantity to be dried, and rain and dew hazards. High-moisture paddy depths range from 1 to 2 in. (2 to 5 cm.); low-moisture paddy depths range from 3 to 4 in. (8 to 10 cm.). As the rice dries, it is raked or otherwise stirred, to expose new surfaces. During rainy periods the rice must be gathered up or otherwise sheltered until the weather is again favorable.
Sun drying commonly causes breakage, regardless of whether rice is unthreshed and dried in swaths and bundles, or threshed and dried on floors. Milling yields improve considerably when the grain is dried in the shade. However, drying time is longer.
There is little correlation between drying methods and climatic conditions, and that the poor milling yields often obtained dispel the idea that drying methods have been gradually perfected to produce highest milling yields and quality.
Regardless of the disadvantages, the small farmer prefers sun or shade drying with natural air because he can utilize the labour of the entire family and avoid fuel and equipment costs. Custom drying is avoided because of the cost. Many farmers find it difficult to join in cooperative drying ventures. Moreover, mechanical drying facilities are widely scattered, if available at all, and transportation of paddy to driers may be difficult.
B. Threshing and Winnowing
Most of the paddy is threshed by beating the cut plants on logs and frames, human treading on the panicles, or driving animals over the plants after they have been laid on flat surfaces. However, these methods require considerable labor, and mechanical threshers of varying degrees of complexity are also used.
Winnowing consists of tossing the threshed paddy into the air and permitting the wind to carry off the chaff. Fans motivated by bicycle-like arrangements or electric power are also used. Pebbles, clods, or other heavy particles cannot be removed by winnowing. Mechanical threshers often include fans and other devices to remove chaff.
The International Rice Research Institute has developed small threshers for " underdeveloped areas.
C. Mechanical Drying
With increased rice production in a number of develop-ing countries, primitive harvesting and drying methods have become inadequate. Technical competence in modern methods is improving in these countries, and there is a growing aware-ness of losses by sun-drying methods owing to cracking; depredations by insects, rodents, and birds; and spoilage after rains. In addition, some of the newly introduced, high-yielding varieties may be planted at any season to produce several crops per year. Some will mature during rainy seasons. Mechanical drying is therefore increasing.
Mechanical drying, as used here, refers to the use of fans to move air (either natural or heated) through wet threshed paddy. A great many drier designs are used, and they may be classified as deep-bed, shallow-bed, and variations of the heated-air driers described earlier in this chapter. There is little bulk transporting of rice with the drier facilities, but the use of augers, belts, and elevators is increasing. Deep- and shallow-bed driers usually use supplemental heat in humid climates, but unheated air is sometimes used when relative humidities permit. Shallow-bed driers consist of screen-bottomed, coverless boxes above warm-air plenum chambers.
A variation of this drier, which dries the rice in stages, resembles a nonmixing columnar drier with a 12-in. distance between screens. The paddy is held in the drier without movement while heated air is passed through the pack to remove about 2% moisture before tempering. A series of these columns is used with a duct system that directs the air to any one of the columns so that paddy is drying in one column while tempering in the other columns. Drying and tempering sequences are continued without rice movement until the paddy is dried. A column may be filled or emptied while rice in the other columns is drying or tempering.
Another variation of the multi-stage system uses 3 to 4 ft. (0.9 to 1.2 m.) deep squat-bed bins with perforated floors, and tempering bins. Paddy is placed in the squat bins, where moderately heated air is passed through the bed for about 6 hr. to remove approximately 3% moisture. The paddy is then transferred to tempering bins, and after 6 to 8 hr. it is returned to the drying bins. This process is repeated until the paddy is dry.
Other types of driers are small versions of columnar mixing and nonmixing driers. Another type is an oscillating screen drier, in which grain passes down almost-horizontal oscillating screens while heated air is passed through the moving beds.
VI. SEED RICE
Rice seed should be well matured and free from hulled or broken kernels, seeds of other varieties, red rice, weed seeds, hulls, and trash. Kernels should be plump and have a high germination, 85% or better. Rice intended for seed is usually harvested at relatively low moisture levels in order to obtain higher kernel weights and avoid immature kernels. Special care must be taken to avoid mold damage at all production, harvesting, and drying stages.
Favorable conditions for maintaining the viability of seeds are those which slow the metabolic rate. For practical purposes these conditions are a low moisture content and a cool temperature. Seed rice commonly is dried to a lower moisture content than is rice sold for milling. Drying to 12% m.c. is adequate for seed stored less than 1 year. If storage might be extended for a considerably longer period, drying to below 10% m.c. is recommended. Occasional aeration with dry, cool air generally improves environmental conditions for seed rice in bulk storage.
It was shown that loss of viability as a result of drying increased with both rice temperature and moisture content. The zone between the temperature at which damage to germination was barely perceptible and the temperature at which germination was totally damaged was narrow at any moisture content. For 25% m.c. rice the damage to germination was barely perceptible at 50°C. (122°F.), but it was complete at 61°C. (142ºF.). For 11% m.c. rice this zone was between 64°C. (147°F.) and 73°C. (163°F.).
A statistical analysis of laboratory-scale rice-drying tests indicated that germination losses were closely related to both air temperature and initial moisture content, but head-yield loss was related only to air temperature. This research suggests that lower air temperatures should be used initially for drying seed rice at a moisture content above 20%. At lower moisture contents, temperatures that preserve milling yields will not hurt germination.
The handling of seed rice in bags has been an effective method of avoiding varietal mixing. A sack drier is used when handling is exclusively in bags. Heated air is blown into a plenum chamber with openings covered by bags of rice. In Texas high milling yields and good germination resulted when a sack drier was operated with a maximum air temperature of 110°F. (43°C.) at an airflow rate of 238 m.3 per hr. per 49.9 kg sack of dry rice. High labour requirements in sack-drier operation have caused many seed-rice producers to abandon this method in favor of a bulk handling system.
Seed rice may be dried by either the deep-bed or multipass method. Careful cleaning of driers and conveying equipment is required to avoid mixing when switching from one variety to another. Labour requirements for such cleaning may be considerable unless the drying unit is specifically designed for easy cleaning and inspection. Some seed producers have found that the best solution is to provide a different drying unit for each variety.
RICE MILLING TECHNOLOGY
I. Introduction
Processes for milling rice remove the hulls and part or all of the bran, and thus provide the consumer with partially or fully milled rice. Development of these processes has occurred at differing rates in different countries. The simple method of hand-pounding in mortar and pestle type equipment is still used in some areas of the less technologically developed countries. In other societies the process has evolved into a complex, highly mechanical series of operations aimed at providing the largest percentage possible of milled unbroken kernels that are as free as possible from seed and other foreign matter.
II. REMOVAL OF FOREIGN MATTER FROM
ROUGH RICE
Dried rough rice is delivered to the mill in truck trailers and railroad hopper cars where it is dumped and weighed on a batch-type scale Fig. 2.10. in the receiving department. The rice is then conveyed to a Carter Scalparator, M-l, for the first of a four-step cleaning operation. (Since the same symbol is used for the Scalparator and an aspirator, the letter "S" in the symbol is used to distinguish the former.) The Scalparator has an enclosed rotating cylindrical "scalping" reel, made from 1/2 X 1/2-in. hardware cloth that removes (scalps) long pieces of straw and other large foreign matter. It also has at least one settling chamber that removes the dust and other fine material by air separation. The weight of foreign matter removed by the Scalparator and discarded varies from lot to lot, but can be as high as 0.6%. The foreign matter removed by the Scalparator is shown being discharged into a container, usually a barrel or a burlap bag. Virtually all mills now pay growers on the weight of rough rice before scalping, thus absorbing scalping losses in their operating costs, but in the past it was customary to scalp before weighing. Rough rice usually goes into storage bins after scalping, but this step can be bypassed and the rice sent directly to the mill.
M-2 is a batch scale that weighs in 100-lb. increments and registers the number of dumps on a remote counter in the miller's office.
M-3, a screener (rough rice rotex), is a diameter sizer that combines horizontal reciprocating motion with a rotary motion. It can have one or more screens; this machine has two. The top metal screen (scalping screen) has 8/64 X 3/4-in. perforations, and large foreign material that does not pass through ("overs") is collected and later discarded. The second, or bottom, metal screen (and screen) has 7½/64-in. diameter perforations.
The overs from the bottom screen of M-3 go to M-4, a carter disc separator (stick machine)-a length sizer with size SS indents for "lifting" the rough rice out of the sticks and straw that were not removed in the first two cleaning machines.
The rough rice now passes over the first of four permanent magnets in the heads flow to remove ferrous metals. Since these magnets are not machines, they are designated by an "x" rather than a number.
The rough rice then flows to another disc separator (seed machine), M-5, with size AC indents in series with size EE indents for removing the weed seeds and shelled grains of rice. The efficient removal of seeds is probably the second most critical operation in rice milling because of the degarding effect of seeds in milled rice. Fortunately, most varieties of seeds that rice mills must remove are shorter in length than the shortest rough rice, because seed diameters span the range of rough-rice diameters. Seed is the only recognized rice defect that can theoretically be completely eliminated in the mill. Seed is becoming progressively less of a problem as the growers use new and better weed killers; it is in the grower's interest to do this, since the presence of seeds usually reduces the value of rough rice.
III. REMOVAL OF HULLS
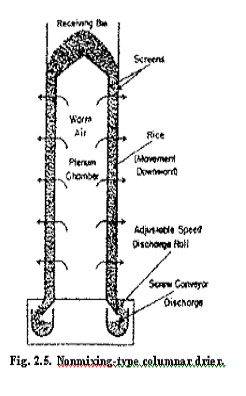
The next machine, M-6, removes the hulls from the grains and is the first in the flow that was designed specifically for rice milling. It is a rubber-roll sheller, or "huller." Referring to Fig. 2.11. a cross-section of a sheller, rough rice gravity-feeds down between rubber-surfaced rolls that are turning in opposite directions and at different speeds. The pressure between the two rolls is regulated by a pneumatic cylinder and can be varied, because different varieties of rice require different shelling pressures; too much pressure will cause excessive breakage and smudging and discoloration of the grain by the rubber. The newer shellers are equipped with automatic controls that will separate the rolls if the rice flow ceases for any reason, thus preventing wear from rolling contact. Depending on the variety of rice being shelled and on the throughput, the rubber on the rolls has to be replaced approximately every 125 hr. The desired minimum efficiency of hull removal is 92%.
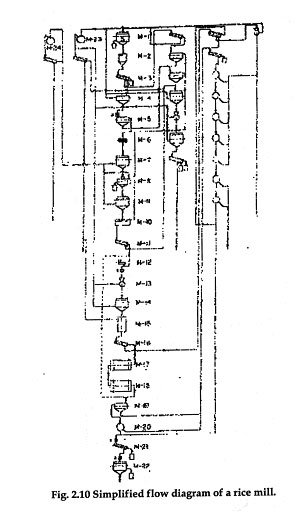
Most mills now use the rubber-roll sheller because it causes less breakage and has a greater efficiency of hull removal than earlier types of shellers. The earlier disc sheller Fig. 2.12 has a horizontal emery-faced wheel revolving on a vertical axis above which is a stationary wheel, also emery-faced. Rough rice flows vertically down through an opening in the center of the stationary top wheel and horizontally out between this wheel and the rotating bottom wheel through centrifugal force. The abrasive action of the two wheels on the rough rice removes most of the hulls, as is shown in the blown-up cross-section.
The next three machines in series in the flow-M-7, an aspirator;M-8, a monitor; and M-9, an aspirator-utilize air to separate the light hulls from the heavier brown rice2. The monitor also has a series of screens to help in this separation.
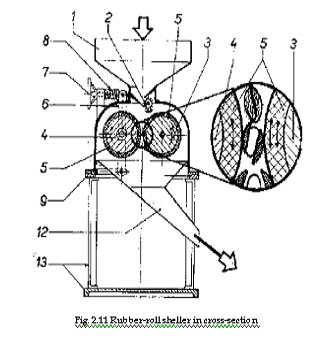
M-10, a gravity separator (paddy machine), separates the unshelled rough rice from the brown rice, and the former is returned to the sheller. The older paddy machines have provisions for adjusting the speed and slope of the table; the newer machines, primarily manufactured in Germany and Japan, also have a provision for quickly changing the length of stroke.
The Rotex, M-11 , removes the smaller broken grains from the unbroken kernels of brown rice, using an 11 -mesh screen. This separation is made because smaller broken grains going through the pearler with the unbroken grains have less bran removed than the latter, resulting in milled rice with more than one degree of milling.
If the desired finished product is brown rice, then machines M-12 through M-18 are bypassed, as is shown by the dotted line. Rice to be milled, however, flows next to the bran-removal process.
IV. REMOVAL OF BRAN
M-12 is a screw-type feeder that adds calcium carbonate to the brown rice at a rate of approximately 1/3 lb. per cwt.; it is not added to some lots that are to be lightly milled. Calcium carbonate is a dry abrasive powder that facilitates the removal of bran by the pearlers.
Some varieties of rice have bran layers that adhere to the grain more tightly than others, and in these cases water at room temperature is next dribbled on the brown rice to soften the bran layer. The water also causes the calcium carbonate to better adhere to the brown rice, with the result that less is needed. It is believed that steam or atomized water would be more effective than a stream of water because either should cause less fracturing of the grains and better distribution of the moisture.
The pearler, M-13, which removes the bran, is the most critical machine in the mill since it causes most of the grain breakage, even when properly adjusted.
The higher the pressure, the more bran removed, specification recognize only there degrees of milling (i.e., amount of bran removed), but the mill described produces four degrees.
Currently, the degree of milling is determined visually by the "pearler man," or miller, so differences of opinion frequently arise. The successful development of such an instrument should take most of the guesswork out of this "black art" area. The ultimate instrument would continuously sample the discharge from a pearler, measure the degree of milling, and, through feedback, control a mechanism that would regulate the pressure on the plate, thereby producing rice with a uniform degree of milling.
It is the industry's opinion that most rice mills should control the pearler operation better than they are now doing. As an example, simultaneous measurements were made on eight production pearlers that were set by a qualified miller to produce the same throughput and degree of milling. The throughput varied as much as 100% from the lowest figure, and the degree of milling varied over almost the full range possible. If the throughput exceeds the pearler manufacturer's recommendation, the percentage of brokens produced increases rather dramatically. There are at least two techniques for better controlling the degree of milling that bear mention, although it should be understood that these refinements are not being used currently in this country. Some mills control the degree of milling by continuously weighing the bran removed by all the pearlers, and' if the rate of removal for a given throughput of rice varies from a preset figure, the pearling pressure of all the pearlers is automatically adjusted by a feedback signal actuating a servo-mechanism. Other foreign mills separate the brown rice going to the pearlers into two sizes, unbroken and large brokens, and the two sizes are pearled separately but simultaneously. As mentioned earlier, this practice should lessen the problem of producing rice with grains having more than . one degree of milling.
It has been proved experimentally that brown rice should be processed in an atmosphere that has a relative humidity equivalent to the equilibrium moisture content of the rice. This phenomenon has also been proved empirically by millers who have noted that the head yield sometimes changes noticeably as the relative humidity in the mill changes appreciably; this usually occurs as the air temperature goes down at night. There was at least one older mill built with a system for humidifying the air in the mill, but it has been torn down.
The mill in Fig. 2.10. has the pearlers in parallel and, in the parlance of the industry, this is a "I-break" system. Other mills have up to three breaks, and some mills have as many as five. The rationale for multiple breaks is to remove only a portion of the bran in each break through reduced pressure, and thus cause less breakage. To quantify the importance of the breakage problem that has been stressed here so heavily, realize that if the mill described could reduce the percentage of brokens produced by just 1%.
The pearler is equipped with a bran-removal system. A blower, belt-driven off the main shaft, forces air into holes in the hollow center of the main shaft, and then out through holes in the portion of the shaft in the pearling chamber. This air picks up the loose bran and carries it through the screen, into a hood, from which it is drawn by another blower that is connected to all pearler hoods. Another purpose of the air flow is to cool the rice, since heat generated in the pearling operation raises the temperature of the grains, inducing stress cracks. The screens must be replaced approximately every 200 hr. because wear holes allow grains of valuable rice to be carried away with the bran.
Although not used by the mill described, machines called whiteners are sometimes used in place of pearlers or in series with pearlers. Their principle of operation differs from pearlers in that the bran is primarily removed by the rotation of an abrasive roller against the grains and, to a lesser degree, by the friction of the outer screen against the grains. The whitener, therefore, loosens the bran essentially through the action of external friction against the grains, whereas the pearler loosens the bran primarily by friction between the grains. Compared to pearlers, whiteners generally cause less breakage, but more breaks are required and the surface of the resultant milled rice is rougher, necessitating additional polishing equipment downstream.
Most of the loose bran is removed by the aspirator, after which the rice goes through a brush. This brush consists of a rotating vertical cylinder to which strips of leather are attached. The milled rice passes down between this cylinder and a surrounding wire circular screen, and the action of the rotating leather strips causes limited additional bran removal. Basically, however, the brush is considered to be another aspirator that removes the loose bran that might be left after the previous aspiration. Some mills are discontinuing use of the brush because it is thought to cause excessive breakage.
V. Sizing of Milled Rice
The milled rice now goes to a single screen where the brokens grains were produced in the pearling operation are separated from the unbroken grains by a 9-mesh screen.
The next machines, M-17 and M-18, are large wooden trumbles that rotate slowly about a horizontal axis, polishing the rice inside by the relatively gentle action of the grains sliding across each other. If the rice has been lightly milled small amounts of glucose and water are added to the rice before the trumbles that the tumbling action will cause the remaining bran to adhere more tightly to the grain. If this is not done, the bran will tend to come off in the subsequent sizing machines and clog them.
A few customers request a rice having a special pearly appearance achieved by adding glucose, water, and talc and then polishing it in the trumbles. To achieve the desired appearance, this rice, which is called "coated" in the industry, must have all of the bran removed before the coating is applied.
If vitamin powder (enrichment) is to be added to the rice, it is applied by a screw feeder, along with water, just before another two trumbles in series. Therefore, all enriched rice goes though four trumbles.
In addition to heads, the following products and by- products are usually produced in the milling operation: second heads, screenings, brewers, bran, and hulls.
Figure 1 also traces the flow of these products and, with the exception of hammer mills, there is no machinery used that was not described in the heads flow. The second heads and screenings are usually blended back into the heads rice, as well be discussed later, while the brewers are usually sold to breweries for use in the making of beer. All rice is always sold for human consumption.
Batch scales connected to remote counters continuously weigh all of the above products, except hulls, as they are conveyed from the mill to storage bins. At the completion of a lot, these scale readings are carefully compared with three separate analyses for heads yield and total yield that have previously been made on that particular lot. These analyses are: the rough rice buyer's, made in the field; the grader's, made when the rough rice arrives at the mill: and the pilot lab's, made by production personnel as the rice starts the milling process. If there are significant variations between any of these analyses and the actual results, immediate steps are taken to determine the reason. Also, during the milling process, hourly samples are taken of the various product flows and comparison made with the above analyses for all defects. A rate-of-flow recorder was recently connected to the heads scale to help the production personnel to reduce variations in the output of the mill.
For the mill described there are six men and a miller on each shift, with four of the men spending most of their time on clean-up. There are two additional men on the day shift whose primary job is to load out the bran and hulls. This mill averages 16 hr. of operation per day, 5 days a week, except for a short down time for maintenance just before the rice harvest each year.
The heads, second heads, screenings, and brewers all go to separate storage bins where they are sometimes mixed, respectively, with heads, second heads, screenings, and brewers from other lots, if the quality and variety permit. If an order specifies head rice, which by specification cannot have over 4% brokens, then it is conveyed directly from the heads bins to one of three separate bagging scales or to the consumer package department bins. For those orders specifying over 4% brokens, a blending operation is necessary. This is accomplished by mixing rice from the belt conveyor running below the second heads and/or screening bins with rice from the belt conveyor running below the heads bins. The conveyor streams are fed together into the boot of an elevator which discharges into an aspirator, and then to a bagging scale. Sometimes as many as five bins are drawn from at the same time in order to get the desired percentages of defects. The discharge from each bin is regulated by a self-powered gravimetric feeder, but a more accurate metering device is needed, hopefully one that would have its own feedback control.
Considering the various combinations of brokens, seeds, and other defects, the mill described has over 50 different milled-rice specifications which are convertible back to at least 20 different rough-rice specifications. Choosing the rough-rice lots to mill for given orders so as to assure the lowest possible rice cost can be quite a formidable task. To-help in this area, the author, in conjunction with outside consultants, recently completed a rough-rice-selection and milled-rice-blending linear programming model that should be of considerable benefit to top management. Properly designed, an L.P. model, which must be run on a computer, always determines the optimum solution to a particular problem. Rough-rice selection and final blending are so complex that it would be highly improbable that personnel working on calculators could ever determine the optimum solution.
At the beginning of this chapter it was said that an "actual" not a "typical," rice mill would be discussed because, to the knowledge of the author there is no typical rice mill. As was mentioned, there are mills that the author pearlers only, and others that have whiteners only; some have one break while others have as many as three. Some mills have only uniflow cylinders as length sizers while others have only disc separators. Obviously, there should be one "best" way to mill a given variety of rice, but much experimental work still needs to be done before it is found.
SOLVENT EXTRACTIVE RICE MILLING
I. INTRODUCTION
Solvent extractive milling of brown rice to white rice is a relatively new rice-processing technology. Its commercialized form carries the trademark X-M. Advantages of the process are found in traditional problem areas of rice milling: 1) loss of edible rice, 2) kernel breakage, and 3) general under-utilization of rice bran. With an X-M milling facility, higher yields of edible rice are obtained, fewer rice kernels are broken, and rice oil is extracted from the bran at the time of milling to produce two co-producis-an edible defatted rice bran and a crude dewaxed rice oil.
The purpose of this chapter is to acquaint the reader with the principles and commercial 'application of X-M technology. An explanation of the concept, the processing techniques used to achieve the aims of the concept, and the characteristics and market potential of the products are presented. Also included is an outline of the procedures used to substantiate the rice-milling yield increases and a: review of the economic data associated with new ventures in X-M rice milling. Because the only operating facility using this process is owned by Riviana Foods Inc., whose subsidiary, Food Engineering International, Inc., holds the primary license, their trade name, X-M, will be used in this chapter in the interest of brevity rather than solvent extactive rice milling. The technology is protected by patents in most rice-producing and rice-consuming nations.
II. THE X-M CONCEPT
The processing of dried rough rice to polished table rice can be separated into four distinct operations. The first is cleaning paddy rice of miscellaneous straw and dirt. This is followed by shelling to remove the hull and to produce brown rice. The third step is to remove the bran layers from the endosperm, which for the purposes of this chapter will be called the bran-removal step. The final operation is grading to remove broken kernels and to prepare the finished milled rice for storage or shipment to purchasers. A by-product stream composed of bran, germ, and rice polish is obtained during the bran-removal step. Occasionally, the polish is maintained as a separate stream.
It is during the third operation, the bran-removal step, that major economic losses are incurred. The common practice of using dry mechanical grinding or frictional pressure machinery to remove the tightly adhering bran layers results in abrading away portions of the starch endosperm to a flour and breakage of many whole kernels owing to excessive pressure and heat build-up. The yields of both whole grain and total rice product are significantly reduced from those potentially available. Additionally, the rice-bran stream undergoes rapid quality degradation due to the enzyme-catalyzed hydrolysis of the oil contained in the bran.
The concept of X-M milling is to perform the bran-removal step under more gentle conditions- in the presence of a liquid hydrocarbon solvent in order to increase the yield of rice, to reduce kernel breakage, and to extract the fatty components from the entire kernel, bran, and germ so that enhanced quality by-product streams of edible defatted rice bran and crude dewaxed rice oil are obtained. Through this, the usual by-product bran stream containing the major portion of the grain's oil is upgraded to two co-product streams with the rice. X-M is, therefore, a substitute or replacement processing technique for the conventional bran-removal step in rice processing. The other processing steps remain unchanged. This relationship of X-M to conventional facilities is desirable because X-M processing can be either incorporated into the construction of new facilities or added into the modernization of existing facilities.
III. THE DEVELOPMENT OF X-M
The concept of X-M has been developed beyond theory into a successful, commercially proved technology. The operation has proved dependable and consistent in the continuous production of high-quality white rice, edible defatted rice bran, and crude dewaxed rice oil.
The attainment of this continuous commercial operation was the result of several evaluation steps.
The basic steps performed in this X-M plant are: a) pretreatment of the brown rice prior to milling, b) milling the pretreated brown rice in the presence of a rice oil/hexane miscella, and c) separation and recovery of the defatted rice bran, crude rice oil, and hexane.
Extensive process-development studies have been conducted from the origin of this operation to the present time. Much of the early developmental work was centered on the resolution of mechanical problems. Many difficulties were recognized, analyzed, and overcome in improving this new technology. Within the wide range of experience and knowledge obtained during this development program were: 1) satisfactory separation of extremely fine bran particles from the liquid stream by the development and installation of an effective miscella-clarification system, 2) improvements in design and operating techniques of solvent milling machines, 3) development and installation of a crude-oil dewaxing system to produce an oil stream suitable for refining to edible oil, and 4) scale-up of the pilot operations to full-scale commercial production facilities.
In addition to the process-development program, the product streams also received study and evaluation. Results show that the X-M milled rice is equivalent to, if not slightly preferred, by consumer comparisons with conventionally milled rice; that crude rice oil can be refined and sold successfully as a retail-quality cooking and salad oil, as well as for typical industrial uses of vegetable oils; that the market for defatted rice bran as animal feed is strong; and that numerous new product prototypes can be produced from the bran and oil.
IV. PROCESS DESCRIPTION
An X-M plant is an integrated facility for the milling of rice and solvent extraction of bran and rice for recovery of defatted rice bran and crude dewaxed rice oil. The X-M process in general must be considered as an industrialized approach to rice milling and, in fact, portions of the plant have the appearance of a small petrochemical operation. All equipment is Class D and protected from explosion hazards.
With this type of equipment, a 24 hr. per day, 7 day per week operating schedule is preferred for efficient, continuous production. However, it is not difficult to start and stop the rice-milling section for short periods independent of the rest of the plant. And, as with conventional facilities, the degree of milling can be adjusted and controlled continuously. Other general characteristics include isolation of the plant from ignition sources and use of safety equipment and procedures typical of oilseed extraction plants.
Most of the process equipment is available from vendors without custom design. Exceptions to this are the milling machines, which are modifications of rotor- and stator-type frictional mills. A patented design has been developed to allow multiple entry of liquid miscella into the milling chamber in addition to seals and enclosures designed to prevent the unwanted escape of vapours and liquids.
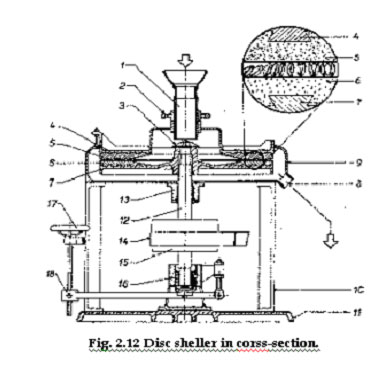
Fig. 2.13. is a simplified flow-sheet of the X-M process.
Brown rice, free from unshelled paddy kernels, is the starting raw material. It is fed to the X-M plant in a continuous stream at a controlled rate. As it enters the plant, it is spray-coated with warm rice oil and transferred to a bin. Rice oil is added, generally at a level of 0.5%. Addition of rice oil and holding from 2 to 4 hr. are two of the key steps in the process whereby the bran layers are softened to facilitate removal.
The next basic element of the process is milling the brown rice to white rice in the presence of miscella. The miscella is a controlled concentration and temperature recycle stream of rice oil and hexane. The treated brown rice is intro-duced into these machines at a determined rate through an adjustable screw feeder. The miscella stream is introduced at several points so that the rice-milling chamber is flooded with moving liquid. Brown rice is milled to the desired degree of bran removal with a one-break system in which the rice passes once through only one milling machine for bran removal. The rice requires about 15 sec. to travel through the milling chamber and is discharged through a pressure plate at the from end of the mill. The back pressure on this plate is adjusted with weights to give the degree of milling desired and typically requires about half that used in conventional dry milling. Bran, germ, and miscella are discharged through the milling screens and slurry and collected in a settler. If desired, additional passes can be made through the milling equipment. This is primarily dependent upon the marketing requirements to be met by the operator.
Passage through a milling machine of this type gives higher yields of milled rice than are obtained by traditional dry-milling methods. Kernel breakage during the bran-removal step is caused by heat and excessive nonuniform pressure. With solvent milling, the miscella acts in effect as a heat sink to absorb and carry away heat generated from friction and as a lubricant to reduce localized excessive pressure gradients. Thus, fewer whole kernels are broken in X-M than in dry milling.
Losses from the theoretical total of clean rice potentially available occur in creation of small particles when the whole kernel breaks, and from gouging of grinding away the rice endosperm in an attempt to remove bran layers present in the irregularities of the rice-kernel surface. With solvent milling, a combination of the softening pretreatment of the bran layers and the lower pressure and lubricating characteristics of solvent milling reduces this gouging effect on the endosperm and results in higher yields of both whole grain and total clean rice than are obtained in conventional milling. Also contributing to increased yield is the flushing action of the liquid to remove the loosely adhering bran particles which otherwise would require contacting forces to set them free. This action also keeps the milling screws from fouling or plugging.
Besides increasing clean rice yields, solvent milling also solves the traditional logistical problems of rice-bran extraction. Immediately upon rupture of the bran layers during conventional milling, fat-degrading enzymes and fatty substrates are brought together. Hydrolysis starts immediately thereafter and a significant increase in the free fatty acid content is observed within the first few hours. This rapid free fatty acid increase has been one of the major detriments to the development of a rice bran-oil extraction industry. The oil obtained by post-milling extraction of rice bran will contain a free fatty acid level of from 5 to 50% and will have a dark brown color. Both factors detract from the value of the oil. Because these defects increase with storage time if no stabilization is employed, the bran may become wholly unsuitable for extraction. With solvent milling, extraction of both bran and rice endosperm is initiated-at the time of milling. Therefore, the oil produced is not subject to enzymatic degradation and is stabilized from free fatty acid build-up. The color also is considerably lighter.
The remainder of the processing steps on the flow-sheet are used for completing the extraction of oil from bran and for recovery of the products and solvent for recycling. Following the flow of rice, this stream is collected from each of the mills and contains a surface coating of hexane/oil miscella, plus some loosely adhering free bran particles. The rice is introduced to a series of vibrating screens in which fresh hexane is used to wash the rice surface. At this point fresh hexane is introduced into the system. After draining of this wash liquid, the rice has a residual hexane content which is removed without thermal shock to the kernel so as to prevent breakage. The rice desolventizer is a vertical tower in which the product cascades from top to bottom through two sections. During this time, the residual liquid hexane is evaporated by the counter-current flow of a warm mixture of inert gases and solvent vapours for transfer out of the X-M plant ready for grading, storage, or packaging.
The slurry of bran, hexane, and rice oil is accumulated in the settler, which also receives the miscella from the rice washing and serves as the surge area for the milling miscella recycle. Extraction of oil from the bran is completed in this settler and the remaining bran-processing steps are used to wash and separate the bran solids from the liquid stream.
A series of two horizontal centrifuges is used to separate the bran and miscella and to wash the bran sequentially with dilute miscella and fresh hexane. The hexane-damp bran cake from the second centrifuge is discharged through a venturi to a superheated hexane vapour recycle stream for desolventizing the bran in a cyclone separator. The dry bran is purged with warm inert gas prior to pneumatic conveyance to storage or a bagging station.
Recovery of the third product, rice oil, is begun with steps to remove residual fine bran solids. The miscella from the first horizontal centrifuge contains some very fine, slow-settling bran solids. It has an oil concentration of 11 to l4%. Solubilized with the oil is the rice wax fraction, which amounts to as much as 3 to 5% of the total fat. This stream passes through a bank of liquid cyclones to remove any remaining larger bran particles. The miscella is then cooled to precipitate part of the wax fraction in the oil. Water and a silicate floccing agent are added to the cooled miscella stream just prior to centrifugation with the high-gravity stacked-disc separator. The fine bran solids and about half of the waxy fraction are removed in this operation. The clarified miscella is then distilled with standard stripper-evaporator equipment to obtain a clear, medium-brown rice oil containing 1 to 2% wax. This stream is properly called rice oil, because it has been extracted from both the bran and endosperm.
The oil is held in a chilled state for 2 to 3 days to allow crystallization of the residual wax. After the holding period, the oil is pumped through a mixing station for a second flocculation treatment. This treatment forms a physicochemical bond with the precipitated wax to weight the particles for easier centrifugal separation. When centrifuged in a typical continuous vegetable-oil refining centrifuge, a clear product oil with less than 0.15% wax is obtained.
Hexane vapors gathered throughout the plant are condensed with water-cooled condensers and returned to a central hexane tank for redistribution. Vapours from the water condensers are vented through a mineral-oil absorption system to trap the residual hexane and allow escape of noncondensibles from the plant.
V. X-M PRODUCTS
The products of X-M processing-milled rice, defatted rice bran, and crude rice oil-have improved characteristics compared to the same products from conventional dry rice milling with or without post-milling extraction of rice-bran oil.
A. X-M Milled Rice
X-M milled rice differs from that conventionally milled in that it has a whiter appearance in the uncooked form and has approximately half the oil content. Sensory testing has shown no differences in the preparation or eating characteristics. it is readily accepted by the consuming public as polished rice because of the elimination of defects such as rancidification and staling by removal of much of the fatty materials. Also, the rice, having been flushed with liquid hexane and warm vapors and processed in enclosed equipment until preparation for storage or packaging, shows significant gains against endemic insect-infestation problems. These two aspects can be of particular significance when only intermediate degrees of milling and partial bran removal are desired.
B. X-M Bran
X-M rice bran is a markedly different product from conventional rice brans. Ii can be characterized as a defatted, free-flowing rice-bran product possessing a bland flavor, pleasant odor, and light color. Chemical analyses show an effective increase in protein concentration derived through elimination of dilution by abraided endosperm and oil removal. Removal of the fat during milling results in a bran product with excellent stability. The composition of X-M bran with comparative typical data for regular rice bran is shown in Table 2.13 and the vitamin and mineral composition in Table 2.14.
When sold as an animal feed, X-M bran sells at prices equal to or slightly above conventional rice-bran feed. The implications of a stable, edible bran stream with an elevated protein content entering the food cycle as more than animal feed are challenging. A limited quantity of X-M bran has been sold at premium prices to food processors for ingredient use. The potential of X-M rice bran in human foods is under continuing study.
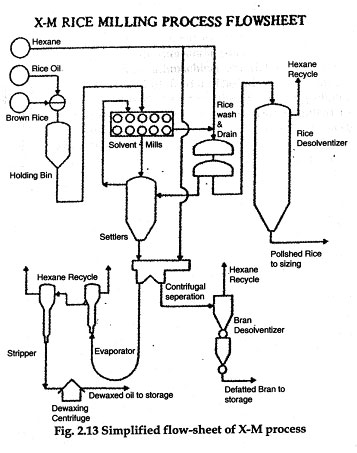
An amino acid analysis of a typical X-M bran is shown in Table 2.15. Variations in the amino acid composition are similar to those found in all bio ogical materials of different origin, maturity, crop year, etc.
Food-product concepts have been developed demonstrating whole X.M bran for bakery use and as a flavor carrier. Enzymatic digests for syrups have been made. Much of the protein in X-M bran can be solubilized with dilute alkaline extraction, and subsequent acid precipitation yields a near-white paste. A protein concentrate of 70 to 75 wt.% can be prepared by this simple process. This concentrate can be used in gel form or dried to a stable film or powder. Prototype food products made from X-M bran protein concentrate included pastas, milk substitute beverages, and confections.
C. X-M Rice Oil
Traditional supplies of crude rice-bran oil have suffered from problems of high refining losses due to high levels of free fatty acids, the presence of the wax fraction, and finely suspended rice solids. X-M processing has greatly reduced these negative characteristics.
Since X-M oil is composed of fatty extractions from bran, germ, and endosperm, the yields of oil with X-M processing are higher than those obtained by post-milling extraction of bran and germ only. The data in Table 2.18 show that X-M rice oil and rice-bran oil have the same general composition.
X-M rice oil has been processed to a quality cooking and salad oil by traditional caustic refining, bleaching, winterizing, and deodorizing techniques. Market. testing has shown this oil to be acceptable to consumers and competitive with major-brand vegetable-oil products. Properties of the processed oil are shown in Table 2.17.
VI. RICE MILLING YIELDS
Rice-milling yields were considered one of the most important net advantages in experimental investigations from the earliest days of the X-M concept. These data demonstrated the higher milling yields obtainable and led to commercialization of the technology.
During problem-solving and optimization activities at the commercial X-M facility in Abbeville, indirect studies showed the commercial facility to be achieving higher milling yields. Definitive studies to document the actual yield increases were made by direct comparative test millings between the X-M facility and conventional commercial facilities. Direct comparison was made because of concern for possible inaccuracies of indirect comparisons when working with a variable raw material such as rice, in which previous handling, as well as characteristics inherent to brown rice, has significant influences on processing performance.
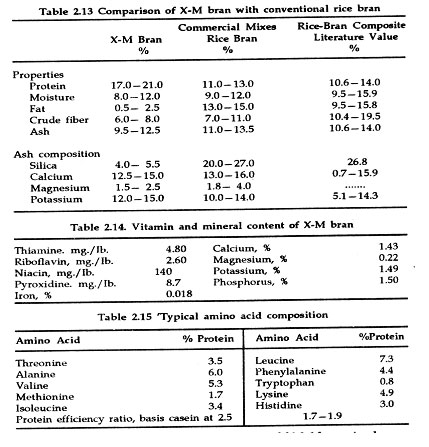
The adjacent conventional bran-removal facilities in the pre-existing rice mill in Abbeville were modernized and equipped to perform modern conventional milling opera-tions on a scale comparable to the X-M plant. Additional equipment arrangements were made such that a given lot of dried field rice could be continuously cleaned, shelled to brown rice, split to feed each processing route, and milled to white rice. In this manner, both processing routes were assured of identical brown-rice raw material which had been subject to the same environment without differences in handling, time of day in milling, or other possible changes.
Scales and samplers were installed to measure total weight and to sample for broken kernels in the brown-rice feed to both conventional and X-M facilities. Similar scales and samplers were installed on the milled-rice streams from both plants. The exact weight fed to each plant, the weights of rice obtained from each plant, and the brokens being fed and obtained from each milling operation were determined for each feed stream.
These tests were made over a period of several months and consisted of 26 separate milling lots of 1- to 4-days duration each. Both long- and medium-grain varieties were milled both for intermediate grade with partial bran removal and for well-milled package-quality products.
The results confirm higher milling yields in terms of both whole-grain rice (from reduced kernel breakage) and total milled rice (from reduction in surface gouging of the grains, which carries endosperm particles out with the bran). The average increase in total yield was 1 to 2 wt.% and the average increase in whole-grain yield was 4 to 5 wt.%. These averages were found for all four types of rice milling, although the individual lot results ranged from little or no increase to exceptionally high increases. Such variation would be expected when working with an agricultural product on commercial operations (Table 2.17).
The yield of dewaxed oil is determined by extraction of the bran from the 17 to 19% level to the 1 % level, plus extraction of the rice endosperm from the 0.7% level to about half that. Some losses are incurred in dewaxing the oil and in miscella clarification as centrifugal underflow. These materials are recovered and redeposited on the bran when the bran product is scheduled for animal food markets. The X-M bran yield is lower than that for conventional milling because of the fatty material extraction, reduced endosperm particles and noncontamination with hulls.
VII. TECHNOLOGY EXPANSION PROSPECTS
The modern trend to vegetable-oil refining in the miscella state has been investigated for the X-M process on a large pilot scale. Successful tests have demonstrated the feasibility of producing a refined, edible oil at the rice-milling site. This concept combines the oil refining and solvent milling processes into a common processing facility.
Input rice from a broad list of varieties of international and domestic origin of the three major types-long-, medium-, and short-grain -have been pilot tested as well as rices of variable quality, e.g., mixed varieties, maturity, and length of storage. All of these rice streams, when presented to X-M milling, have milled satisfactorily. Especially encouraging are observations on improvements in low-quality rices.
Radical machine designs have been successfully pilot tested and patent applications are pending.
Grains other than rice have been pilot milled to remove their bran layers, seed coats, etc. By this process, high-quality specialty cereal products and oils have been produced and are used for study of their compositional and functional properties.
RICE STORAGE
Rice and other cereals supply food for man in a form that can be preserved for long periods of time. This ability to maintain a constant food supply in a permanent location provided the basis for man's development from a pastoral nomad to a dweller in the modern urban communities of our technological civilization. Despite the many advances in food preservation, storage of cereal grains remains the major means of providing a uniformly and constantly available supply of our basic foods.
The motivation that led to the development of methods for grain storage has passed to us unchanged through recent millenia. The reasons for storage and the general principles that apply are basically the same for rice as for other cereals and for most seed crops. Briefly, storage is intended to protect rice as well as other seed crops from the weather and from all types of pests, and to prevent or delay changes in nutritive values or loss in quality. The basic principles of grain storage are relatively simple. First, the product to be stored must be conditioned to a stable state in which respiration of the seeds and of associated microorganisms are reduced to a minimum. Secondly, it must be placed in a container or structure that will maintain a suitable environment and prevent or restrict the entrance of insect and animal pests. Last, the grain must be manipulated, if necessary if necessary to maintain a good condition throughout the storage period.
With thousands of years of experience to draw on, it might be assumed that the principles of successful storage would be universally known and practiced. Unfortunately, lack of knowledge and failure to use the information we now have result in large annual losses of grain or of its economic and food value. Estimates of annual losses during storage have run as high as 20% or more in some underdeveloped countries. Since many storage losses are not recognized, and recognized losses are often impossible or uneconomical to measure accurately, the actual cost of storage losses is not known. In a world with population rapidly exceeding the available food supply, it is increasingly necessary to husband existing food resources and to plug all the gaps in our present technology.
Losses in storage are a direct result of the activities of various macro- and microorganisms made possible by man's failure to understand and follow sound storage principles and practices. Losses caused by insect and mite infestation and by the depredations of large animals such as rats, mice, and birds are usually obvious and generally are recognizable; therefore, the means for control of such pests are generally known and practiced by all reasonably competent persons or organizations storing rice. Losses caused by the activities of microorganisms, are less understood and often unrecog-nized. The first part of this chapter is concerned with the microorganisms most commonly causing deterioration of rice in storage, particularly the fungi. This leads, in section III, into modern storage technology and the design of storage structures. The chapter closes with a study of insect and pest control.
I. DETERIORATION OF STORED RICE BY FUNGI
A. Fungi Associated with Rice Deterioration
Typical fungal deterioration of stored grain was described in 1909, but only since 1940's and 50's has the importance of the problem become recognized. The introduction of combine harvesting of rice, as with all other small grains, has led to an increased awareness of this-problem.
The fungi associated with rice are arbitrarily divided into two groups and arbitrarily designated as either "field fungi" or "storage fungi". This practice is also common with other cereal grains. Field fungi invade the seed primarily during development or maturation preceding harvest. "It's common to isolate field fungi... from close to 100% of freshly harvested, surface disinfected kernels of sound ... rice of the highest grade". In stored rice,field fungi die gradually or rapidly, depending on moisture content and temperature and on the competition of other fungi, especially the storage fungi.
Storage fungi are post-harvest invaders. Many common species can invade and grow in seed with little or no free water. It was found that many of the common species invade stored grain with moisture contents of 13 to 18% in equilibrium with relative humidities of 70 to 85%. However, some species can invade and compete with field fungi, growing rapidly and causing loss in quality in seed with moisture contents above 18%. Since rice usually is harvested with a moisture content of 18 to 22%, drying or conditioning until the moisture is below 18% must be done with dispatch. Propagative units of common species of storage fungi are found on the surface of almost every kernel of rice after harvest. It is assumed that these fungi grow and sporulate on dead leaves and other nonliving organic materials in the rice paddy and that they are disseminated by the combine in the process of cutting and threshing.
The storage fungi are largely responsible for deterioration during storage. However, the exclusive application of this simplistic nomenclature to fungal-deterioration problems should be avoided. Fungi invading prior to harvest may continue to grow within infected seed as long as moisture and temperature are favorable. Conversely, factors such as over-maturity and lodging may lead to invasion of kernels by storage fungi while they are still on the plants. However, the terms are useful and definitive.
Although the fungal flora of rice has been studied complete listing of all fungi that have been found associated with rice has not been assembled. They found that Curvularia and Fusorium species were among the principal genera isolated from hulls of all varieties. The most common genera of field fungi are Helminthosporium, Nigrospora, Fusarium, Curvularia, and Alternaria. In general, field fungi are either pathogenic or semi-pathogenic.
The storage fungi are generally saprophytes or facultative parasites and are principally species of the genera Aspergillus and Penicillium. Rhizopus and Mucor also may develop in rice in storage. Research reported to date indicates that most active storage fungi in rice has been divided into 18 groups. Series of two of these groups, the A. glaucus and the A. flavus groups, appear to constitute the major fungal species that invade stored rice. Other groups often isolated include the A. restrictus, A. niger, and A. candidus groups. Although species of Penicillium are almost universally found associated with rice, they do not commonly invade a significantly high percentage of rice in commercial storage.
B. Effect on Economic Value
The most obvious economic loss associated with fungal deterioration during storage is loss of grade in white milled rice. Rice may be downgraded for; 1) damaged kernels, 2) heat-damaged kernels, 3) change in general color, and 4) musty or moldy odour. All of these factors are or may be caused by the activities of fungi during storage, damaged kernels is defined as "... kernels and pieces of kernels of rice, which are distinctly discolored or damaged by water, insects, heat, or any other means...." The standards do not specifically mention fungi as a cause of damaged rice. It is important that fungi be recognized as the principal cause of discolored rice kernels, since a knowledge of basic factors is the surest way to promote understanding of the need to practice proper storage techniques for controlling excessive damage.
Much of the damage, such as discolored kernels, caused by fungi occurs prior to harvest as a result of infection of the developing or immature seed by field fungi. However, the activities of field fungi may continue if rice is not adequately conditioned shortly after harvest. Such fungi may persist in an inactive state in dry rice, ready to resume growth if conditions of moisture and temperature should become favorable through rewetting or the accumulation of moisture around hot spots or through moisture migration and condensation in limited areas. In most lots of rice, however, field fungi tend to die out in storage. The rate of their disappearance varies from lot to lot. This rate tends to correlate inversely with the increase in storage fungi, suggesting that they may actually be killed or perhaps only hidden by the greater vigor of the storage fungi under conditions of storage. Most field fungi produce pigmented materials when growing in rice. These pigments are usually dark brown or black, although a red discoloration is not uncommon. Dark brown or black discolorations caused by Helminthosporium oryzae are often found in high percentages of the kernels, the mycelium was found in kernel endosperm.
Storage fungi also have been shown to cause discoloration, i.e., orange stain by Penicillium puberulum and "yellowish" by P. islandicum. All such discolorations are a direct result of elaboration and deposition of pigmented compounds by the fungi. Since most fungi produce colored compounds of some kind under the proper conditions, few if any fungi can be definitely eliminated from the list of possible causative agents.
The most common discolorations, dark brown to black and sometimes reddish spots covering part or all of the kernels, are often called "peck" or "pecky kernels , and have been associated with stinkbug damage. In our laboratory, we have developed typical peck in the absence of stinkbugs and believe that the stinkbug is a vector introducing the fungi that are the direct cause of the discoloration. Damaged kernels or peck often break during milling of white rice, and are thus eliminated from the head rice. Naturally, this breakage reduces head-rice yield, and thus the value of the rice. When parboiled, such kernels are strengthened through the gelatinization of the starch which, upon redrying, strengthens and toughens the kernels. Unfortunately, the discolorations are usually intensified and often diffused throughout the kernel. Such rice must be removed from the final milled product by expensive sorting, introducing another economic-loss factor.
Heat-damaged kernels are defined as "dark amber to brown kernels." They closely resemble a "dark parboil." Their presence in rice is considered evidence that the rice has undergone deterioration. The exact cause of "heat damage" has not been clearly established, as it can occur without a measurable increase in grain temperature. When spontaneous heating does occur, heat-damaged kernels are also usually found, microorganisms growing in organic materials can cause an increase in temperature. It is further stated that : "Where insects have been eliminated, . . . the sole cause of heating is the growth of storage molds (fungi) was found in the grain." significant correlation between the percentage of heat-damaged rice in experimental storage and an increase in storage fungi. It is now generally accepted that heat damage is usually caused by microbiological agents (storage fungi).
The colour of rice in mass increased with time in storage, moisture content, and activity of storage fungi. Rice stored at moisture contents above 14% (wet basis) turned from white, to cream, to rosy, to gray, to brown with increased moisture content and time in storage. The actual mechanism responsible for this colour-change is not known, but apparently it is directly related to the level of fungal activity.
It was found that sterilization and incubation under anaerobic or evacuated conditions prevented discoloration, although pronounced discoloration was induced in nonsterile moist rice within 48 hr. after incubation in a normal atmosphere at 25° and 30°C. They concluded that the discoloration induced in their experiment was primarily microbiological in origin.
Musty or moldy odours are not allowable in rice above Sample Grade. Fungal activity must be quite high before these odours develop. They often are associated with sporulation of some of the storage molds.
C. Effect on Nutritive Value
Actively growing fungi cause many compositional changes in rice. In common with other grains, increases in free fatty acids and changes in the proportions of reducing and nonreducing sugars of rice have been associated with fungal activity. Not all of these changes may be detrimental from the standpoint of nutritive values. The growth of fungi may actually increase the nutritive value in some cases by producing some vitamins, growth substances, essential amino acids, and other such substances, when a total or partial deficiency may have existed. However, respiration of the fungi results in loss of dry matter and hence a loss in total available food. Moreover, there are so many strains and species of fungi interacting with each other and with an infinite variety of environmental conditions that it is not possible to predict what kind of changes will occur. The biochemical changes produced by even the most common and prevalent fungi in rice under controlled conditions have not been studied extensively and it is evident that knowledge in this area is extremely limited. Therefore, it is safer to assume that fungal activity in stored rice has a detrimental effect on the nutritive quality of the grain-unless and until proof to the contrary is established.
D. Mycotoxins
Some metabolites of fungi that grow in the rice have been shown to be detrimental, or even toxic, to humans and other animals. High incidence of beriberi was associated with the prevalence of moldy rice induced by a long hot rainy season. It was reported that Penicillium sp., later identified as P. citreo-viride Biourge, isolated from yellowed rice in storage was experimentally shown to cause a nervous disturbance in rats leading to death. The studies resulted in the discovery and identification of two toxic compounds from P. islandicum Sopp. These compounds, luteoskyrin and a chlorine-containing peptide, were shown to cause liver injury and liver tumors in rats.
There are several possible reasons: 1) Rice is generally handled, conditioned, and stored with enough care to ensure overall high quality, and very little moldy rice reaches the consumer; 2) the average consumption of rice by humans or of rice products in animal feed is low enough that moderate contamination might not cause recognizable symptoms;
3) isolated cases of disease or death may have occurred with no association with the causal agent; and 4) some disease problems might result from an accumulative effect over years of consumption of very small amounts of these compounds. Since many of the fungi developing in stored rice are common to all cereals and to other seeds and their products, rice may be a minor source of such toxins.
Discovery of a series of toxic metabolites produced by Aspergillus flavus was triggered by the outbreak of a disease that killed thousands of turkey poults. Subsequently these compounds, named "aflatoxins". were found to be extremely potent carcinogens, capable of causing hepatomas in many species of warm-blooded animals. For the rice industry aflatoxin contamination presently appears only a potential threat, one being generally held in check by the use of adequate technology in handling, conditioning, and storage.
Species of the A. flavus group constitute a universal and significant part of the fungal flora of stored rice. We have found that propagative units (conidiophores, sclerotia, strands of mycelium) of these fungi are present on almost every kernel of rough rice by the time the rice is put in storage. Moreover, actual invasion of individual kernels often occurs in a high proportion of the kernels. In a recent survey of rough rice from commercial sources, we found invasion by species of the A. flavus group that ranged from 0 to 90%. Rice has been shown to be an excellent growth-supporting substrate for species of the A. flavus group and for the production of aflatoxins. Moreover, it was found that 94.4% of 284 randomly selected strains of the A. flavus group isolated from the rice samples were capable of producing aflatoxins. More than one-third of all the strains tested were considered highly toxigenic storing high moisture rough rice Under aeration. It was found that rice aerated at a rate of 0.3 c.f.m. per cwt. was significantly contaminated with aflatoxins within 2 days of storage. The concentration of toxins exceeded 800 p.p.b. after 20 days' storage. Infection by species of the A. flavus group also increased rapidly, with as many as 98% of the kernels invaded in the samples after 18 days.
Doubling the airflow rate greatly decreased both the rate of invasion by A. flavus and the rate of accumulation of aflatoxins in other lots.
However, subsequent studies have been more favorable. It was shown that most of the aflatoxin in contaminated rough rice was located in the bran and outer layer of the kernel. Ordinary milling procedures, therefore, can be expected to remove most of the toxins. Furthermore, subsequent surveys, not yet published, indicate that the prevalence of invasion by toxin-producing strains of A. flavus and the associated development of aflatoxin contamination vary considerably from year to year. Increasingly, the evidence indicates that aflatoxin contamination in rice is on the average much less than was found in the survey.
However, there is no room for complacency. All factors necessary for the development of severe aflatoxin contami-nation are present in rice: 1) Rice provides an excellent substrate for aflatoxin production; 2) the causal agent is always present and may be found on rice in large numbers; and 3) when harvested, rice is usually at optimum moisture content and temperature for rapid development of the causal agent. It is therefore essential that the industry, from producer to processor, follow the best possible practices in conditioning, handling, and storage of rice. Not only can aflatoxin or other mycotoxin contamination be prevented but, perhaps of greater economic importance, quality will be maintained.
II. FACTORS INFLUENCING DETERIORATION
Moisture content is the most important factor affecting the behavior of grain in storage, also temperature, general condition of the gram, oxygen supply, storage duration, and the presence of molds (fungi) and insects are factors affecting keeping qualities. The combined effect of all these factors, within the ranges encountered in storage, is seen primarily in the growth and metabolic activity of the fungi. Although insects directly damage grain through penetration and consumption of kernels, they also influence the activities of fungi. Serving as carriers and vectors of propagative units, they also increase available moisture and raise the temperature, thus fostering and stimulating increased fungal activity.
That moisture content is the most important environmental factor which cannot be denied. Fortunately it is also the factor most easily controlled. There have been many studies to determine the interrelation between moisture and the storage life of rice. Because of interactions between moisture content and other factors, all such studies must be interpreted on the basis of the particular conditions of the study. All of the factors listed-and in addition, variety, grain type, and source-should be considered.
The moisture content of rice going into storage is readily controlled by drying. If the moisture content is maintained at a uniformly low level, rice can be stored for several years with little deterioration, even when other factors are unfavorable. Insects infecting stored rice prefer grain with a fairly high moisture content.
Research in moisture relations in stored grains emphasizes the equilibrium relative humidity of the interstitial air in the grain mass rather than actual moisture content. It may well be that the relative humidity has the greatest effect on the availability of moisture to micro-organisms. The actual moisture content of grain in equilibrium with a given relative humidity depends upon whether equilibrium is reached by absorption or desorption. This effect is called "hysteresis", to affect moisture content of rough rice over a range of relative humidities from 10 to 90%. As an example, he found that rice reaching equilibrium with a relative humidity of 75% at 25°C. by desorption had a moisture content of 14.1%, in comparison to 12.6% when reaching equilibrium by absorption. Rice is usually stored as rough rice because of its greater stability in storage. Thus, the lower equilibrium moisture content of the glumes probably aids in the protection of the kernel by acting as a barrier to the penetration of fungi.
Temperatures below 25°C. are preferable, since low temperatures usually decrease the activity of most storage fungi. However, some fungi grow well in the range of 5° to 10°C. Many Penicillium species grow at low temperatures in the presence of sufficient moisture.
The speed of chemical reactions, including those causing deterioration of rough rice, increases as temperatures increase. A cool storage environment will always be better than a warm environment when other factors affecting storage are equal. Rice, if maintained at a cool temperature in storage, can be stored at a higher moisture content than would be safe at a higher tempera-ture. It was shown that about 20% of the rice kernels were invaded in 45 days in storage at 14.2% moisture content and 24°C. Such high levels of invasion could be very dangerous if some other factor were to change enough to favor the growth of these established infections. Most operators of commercial storage facilities agree that a moisture content between 1 1 and 12% is the preferred range for safe storage.
It is fairly well known that good quality clean grain stores better than poor quality grain, though the reasons are not completely understood. However, poor quality but dry grain often contains well established but dormant fungal infestations that can quickly become active when environmental conditions become favorable. To store rice that is of poor quality, it should be dried even more than good quality rice, or measures should be taken to assure that it is maintained at a cool temperature.
III. STORAGE TECHNOLOGY
A. Rice Storage Structures
Several materials have been used to build structures for storage of rough rice. Any of these are satisfactory if their use results in a building that keeps grain dry, cool, and free of pests. Steel, concrete, and wood are the most widely used.
Steel bins are of many shapes and sizes. These include Quonset, rectangular, and round bins. Bins as small as 800- to 2,500-cwt. capacity are common at commercial driers when rice is stored on an 'Identity-preserved" basis. When rice is commingled, larger bins are advantageous. Rectangular steel buildings, approximately 40 to 60 ft. wide and 120 ft. long, are often used for storing large lots of rice.
Reinforced concrete is the building material most often used in constructing rice storage bins taller than 60 ft. The structures are tight and durable, but their initial cost is high and they cannot be easily adapted for other uses at times when they are not needed for storing rice.
Because of low initial cost, wooden structures are used frequently for storing rice. Extra attention is needed to construct wooden bins tight enough to prevent seepage of water from driving rains and to hold insecticides or fumigants. Metal linings and flashings are needed to exclude rodents.
Storage structures are classified as "flat" or "Vertical", depending upon their height in relation to the width or diameter. Vertical bins have a height greater than their width or diameter, whereas flat storages have a height less than their width or diameter. Vertical storages often have hopper bottoms for unloading by gravity. Power shovels or sweep augers are needed to unload flat bins mechanically.
B. Turning
Turning is accomplished by moving rice from one bin to another periodically with conveyors. This practice is beneficial in several ways. Random mixing of rice from different parts of the bin is helpful in breaking up hot spots and isolated areas where high-moisture rice may be present owing to moisture migration. If turning occurs during cool weather, some overall cooling of the stored rice results. Turning provides the warehouseman an opportunity to inspect the rice and to take corrective measures before damage is extensive. But there is considerable shrinkage during each turning operation. The operation is time consuming and costly when shrinkage, salaries of workmen, and wear-and-tear on conveying equipment are evaluated.
C. Aeration
Many of the benefits of turning can be accomplished by aeration. This has been defined as the moving, of air through stored grain at low rates (generally between 0.1 and 0.2 c.f.m. per cwt.) for purposes other than drying, e.g., to maintain or improve its value. Aeration is far more effective for cooling rice than is turning. Since the rice is not moved, shrinkage due to rubbing of fine material from rice hulls does not occur. Aeration can be used to equalize storage temperatures and to remove hot spots and odours from rice while in storage. An aeration system may also be used to distribute fumigants through stored rice.
The primary benefit of aeration is cooling. The extent of cooling depends upon climatic conditions. Operating aeration equipment only during the coolest weather allows the rice temperature to be kept well below the average ambient temperature at any time of the year. This minimizes mold growth, insect activity, and respiration of the seeds. Aeration generally results in slight additional drying, which reduces susceptibility to mold and insect damage.
Aside from the cooling action that may . take place, aeration equalizes temperatures throughout a mass of stored grain. Unequal temperatures are known to be a cause of moisture migration. When this occurs, pockets of high-moisture-content grain can develop over a period of time even though the grain went into storage at a uniform and safe moisture content.
Aeration can remove odours from stored rice. Sometimes this may be done with a few air changes; at other times the odours may be persistent and require longer aeration. Aeration can be used to distribute fumigants through a mass of rough rice and afterwards to remove the toxic vapors. This is discussed in more detail in the section about stored-grain insects.
D. Aeration-System Design
The first step in designing an aeration system is to select a suitable rate of airflow. This decision depends on the primary purpose of the system and to some extent on the dimensions of the storage structure. An aeration system used only to cool dry rice does not require as high an airflow rate as one used to maintain the quality of high-moisture rice or for supplementary drying by cooling rice between drier passes. The airflow rate selected for a deep bin of rice must be relatively low, because the power requirement for a given airflow rate in a deep bin compared with a shallow bin of equal capacity increases faster than the square of the ratio of the two depths.
The time required to move a cooling zone through a bin of rice depends upon heat-sensible exchange between the rice and air and also upon cooling owing to evaporation of moisture from rice. Both are functions of airflow rate Fig. 2.6 shows estimated cooling times for different airflow rates. One curve shows the hours of fan operation required for cooling undried rice at a fairly high temperature following a drier pass. The other curve shows a longer cooling time required for dry rice when only a small amount of moisture is evaporated.
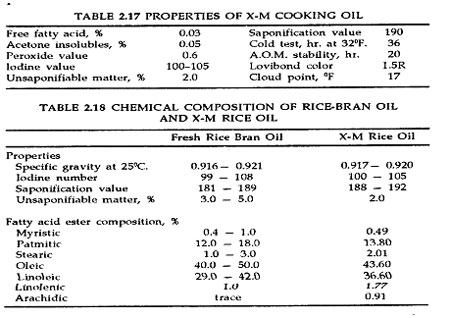
Table 2.12 lists some of the considerations for selecting an airflow rate. Increasing the airflow rate by ten times (from 0.125 to 1.25 c.f.m. per cwt.) increased power costs by seven times for an equal amount of cooling. An airflow rate as low as 0.125 c.f.m. per cwt. is suitable when the purpose of the system is for cooling dry rice during winter months or for equalizing temperatures during other months.
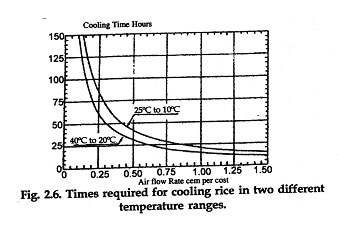
When the primary purpose of the aeration system is to improve storage conditions for undried rice, a higher airflow rate is needed to prevent spontaneous heating. If the primary purpose of the aeration system is for supplementary drying by cooling rice, following a drier, pass, a relatively high airflow rate is needed in order to schedule consecutive drier passes at daily intervals or more often.
The device through which air is introduced into or exhausted from a rice bin is usually a round or half-round perforated duct. Tight-wall ducts with one side open and facing downward are also used for this purpose. The inverted V-shaped channel is one kind of tight-wall duct frequently used. Two areas of a perforated duct the cross-sectionl area and the surface area, are important for aeration-system design. The cross-sectional area of a duct in a vertical bin should be large enough so that air velocity does not exceed 2,000 ft. per min. In a flat bin, where even air distribution is more of a problem, the maximum air velocity within a duct should not exceed 1,000 ft. per min. The surface area of a perforated duct should be large enough so that the velocity of air either entering or leaving the duct does not exceed 30 ft per min. in an upright storage and 20 ft. per min. in a flat storage. The same recommendations apply to the area of opening in a solid- wall duct.
The supply pipe between the fan and a perforated duct is considered to be properly sized when the cross-sectional area is large enough so that the air velocity does not exceed 2,500 ft. per min. A multiple aeration system, in which a single fan supplies air for two or more perforated ducts, requires a branch take-off from the main supply pipe for each perforated duct in the system. A branch take-off should be installed at an acute angle to the main supply pipe so that the direction of the air stream is not changed abruptly. The main supply pipe may be reduced in size progressively at locations beyond each of the branch take-offs; however abrupt changes in pipe size should be avoided. When an elbow must be included as part of a supply pipe, a centerline radius equal to at least 1.5 pipe diameters is recommended.
Either axial-flow or centrifugal fans are used for aeration. Axial-flow fans are apt to be used for aeration of flat storage bins located at a site where noisy operation will not create a nuisance. If quiet operation is essential or if the fan must work against a high static pressure, a centrifugal fan is used.
The fan should be selected on the basis of performance ratings supplied by the manufacturer. A performance rating specifies the volume of air delivered by a fan at various rotation speeds and static pressures. The static pressure can be estimated when the height of rice in a bin is known and a suitable airflow rate has been selected. This static pressure must take into account both the pressure drop in the rice and pressure loss in the aeration system.
This graph is for loose fill of a long-grain rice variety. Some degree of packing occurs as a bin is filled, which increases the pressure drop for any given airflow rate. A packing factor, based on the judgment of the designer, is used to allow for packing by increasing the pressure drop for loose fill by 10 to 50%. The pressure drops in medium- and short-grain rice varieties are some-what higher than for long-grain varieties for the same airflow rate.
In addition to the pressure drop of air traveling in a vertical direction through the depth of rice, allowance should be made for pressure drop of air having a radial direction through rice in the immediate vicinity of the perforated duct. The pressure drop for 1-ft depth at the velocity of air entering or leaving the perforated duct is sometimes used to estimate this pressure drop.
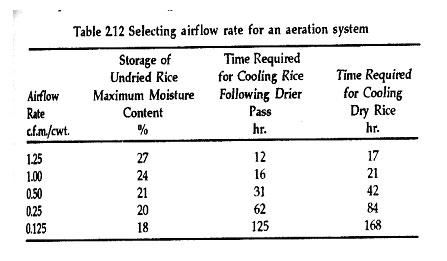
The pressure drop through the supply pipes and the remainder of the system depends upon the distance from the fan to the most remote part of the system as well as on the size of the pipe, and number of elbows, reducers, and junctions.
When these are sized according to recommendations, a friction loss of 0.5 in. (water gage) for each 100-ft. run of duct is a reasonable estimate of pressure drop through the supply pipe. When a more exact estimate is needed, the reader is referred to an engineering handbook such as Heating, Ventilating, Air-Conditioning Guide. Fig. 2.8 estimates fan-horsepower and static-pressure requirements for a range of airflow rates and bin depths. The basic pressure drop for loose fill in Fig. 2.7 was increased by 40% to allow for packing. The radial pressure drop through rice immediately surrounding a perforated duct was assumed to be constant at each airflow rate, and 0.50 in. (water gage) was allowed. The same amount was estimated for the drop due to friction in the supply pipe and other components of the system. Thus, a total of 1.0-in. static pressure was added to the vertical pressure drop in the rough rice. A static efficiency of 62.8% was assumed in calculating fan horsepower. The simplified formula was:
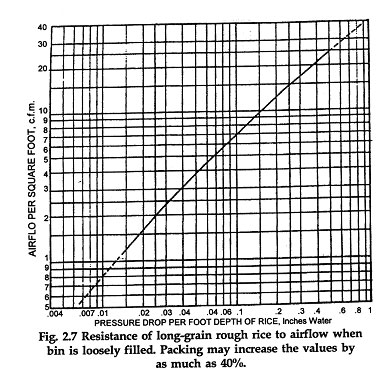
E. Measuring Airflow
The most common method of determining the air distribution and the average rate of airflow is to measure the static pressure at different locations within a bin of rice. One way to do this is to insert into the rice a length of small-diameter metal pipe having a perforated point. The top end of the pipe is attached to a manometer through a-length of flexible tubing. The static pressure at the end of the probe displaces the colored liquid in the manometer which is calibrated in inches, water gage. Pressure drop per foot is calculated from two or more readings at a known vertical distance apart.
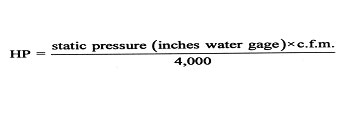
Pressure drop per foot is referred to a graph Fig. 2.7 to obtain the airflow per square foot in a vertical column. The airflow rate can be computed from the airflow per square foot if the depth of rice is known. This method often provides an airflow rate higher than the actual value unless a correction is made for packing.
A vertical bin may be probed -at a single location in the cross-section of the bin. A number of readings should be taken at known vertical distances apart. An average pressure drop per foot is obtained from these readings. When the depth of rice is known, the weight of rice in a vertical column 1 ft. square extending from the bottom to the top of the bin is computed by using a nominal 36 lb. of rice per cubic foot. Airflow in c.f.m. per square foot is divided by the hundredweights of rice in the vertical column to estimate airflow rate in c.f.m. per cwt. Total airflow can be estimated by multiplying the c.f.m. per square foot by the cross-sectional area of the bin.
Flat bins should be probed at several locations to estimate the average airflow rate. Two readings should be made at each location, a known vertical distance apart and both should be well below the top to minimize the effect of a non-level surface. The depth of the pile should be measured at each probe location. Airflow rate is determined for the vertical column at each probe location. The average airflow rate for the flat storage is the mean of all locations where the bin was probed.
The total airflow delivered by a fan can be determined by several methods. Total airflow in a supply pipe can be measured by surveying the cross-section with a Pilot tube. The total air exhausted by a fan can be measured by taking a number of readings with an air-velocity meter to obtain an average velocity. For total air delivery, this velocity is multiplied by the cross-sectional area of the fan's exhaust. Total airflow can also be determined by reference to the fan manufacturer's performance tables when a fan's rotation speed and the static pressure are known. Sometimes these methods are more reliable than determining airflow by probing in rice; however, they do not provide information about air distribution. Whenever possible, airflow should be estimated by probing into the stored rice to obtain the distribution pattern, and the total air supplied by the fan should be determined by another method.
F. Operation for Dry Rice
The operating procedure for an aeration system varies with the primary purpose, the storage period, and climatic conditions. Dried rice to be stored 9 months or less after harvest should be aerated periodically when air temperature is at least 6°C. below rice temperature. Once started, aeration should be continued until the entire mass has been cooled. The overall effect of aeration when air temperature is somewhat colder than rice temperature tends to be a reduction in moisture content, even though the relative humidity may be high part of the time. If all the aeration occurs when the relative humidity is high, a zone of high-moisture rice develops at the location where air first contacts rice. With airflow rates commonly used for aeration, this zone will not penetrate far during a short-term storage period. When the bin is unloaded, any high-moisture rice will be blended with a much larger volume of dry rice.
When rice is carried over one or more summer seasons in storage, the aeration procedure should be changed to avoid operating the fan during periods of high humidity. The fan should be operated periodically when the air temperature is at least 6°C. below rice temperature and when the relative humidity is low enough so that moisture will not be added anywhere in the mass of rice.
Some means of measuring temperatures in a bin of rice is a valuable tool to monitor the progress of a cooling zone while a rice bin is aerated and to indicate that the storage environment is stable at other times. Rough rice is a poor conductor of heat, so the temperatures within a bin of rice are not subject to abrupt changes. A rapid rise in temperature in some particular location indicates heating that might be caused by a pocket of high-moisture rice or activity of stored-grain insects.
If a hot spot is detected in a storage bin, the rice should be aerated continuously, regardless of the ambient air temperature and relative humidity, until the spot has disappeared. Frequently, rice in parts of the bin remote from the hot spot will be warmed. A stage of cooling should follow the removal of a hot spot as soon as the air temperature and relative humidity are suitable. The development of a hot spot indicates that storage conditions are unsatisfactory. After a hot spot has been dissipated, temperatures should be observed at frequent intervals to assure that the hot spot does not recur. If this happens, measures including turning, fumigation, or additional drying may be necessary.
G. Operation for Undried Rice
Continuous operation of the aeration system is required when high-moisture rice is placed in bulk storage temporarily and drying is delayed. Tests on storage of high-moisture rice provided a means to predict safe storage time based on the allowable percentage of damaged kernels. Assays for aflatoxin in rice samples from these storage tests indicated that samples graded No. 1 seldom contained more than a trace of aflatoxin.
The safe storage time for rice below 16% moisture content is much longer than for 18%. Both a long- and a medium-grain variety were maintained at grade No. 1 through 6 months' storage when aerated continuously at a rate of 0.31 c.f.m. per cwt.
IV. PEST CONTROL
A. Stored-Grain Insects
Some 30 species of insects have been found infesting stored rice and rice products, but only a few of these are a serious menace to rice in good condition. The most destructive insect pests of rough rice are the lesser grain borer, Rhyzopertha dominica Fabricicus; the rice weevil, Sitophilus oryzae Linnaeus; and the Angoumois grain moth, Sitotroga cerealella Olivier. These pests bore into and at times completely destroy rice kernels. The lesser grain borer and the Angoumois grain moth are capable of entering the endosperm through the hull, but the rice weevil attacks only grains with broken hulls or with hulls that failed to close properly after blooming. Other species feed on the surface of kernels, on broken grains, and on grains damaged by boring insects. These include the cadelle, Tenebroides mauritanicus Linnaeus; the sawtoothed grain beetle, Oryzaephilus surinamensis Linnaeus; the flat-grain beetle, Cryptolestes pusillus Schonherr; the Indian meal moth, Plodia interpunctella Hubner; the almond moth, Cadra cautella Walker; and the rice moth, Corcyra cephalonica Stainton.
Damage to rough rice from insect infestation results in shrinkage from consumption of rice as well as damage from heating. Active insects generate body heat and give off moisture, stimulating the activity of the microflora. Both are absorbed by surrounding rice and a hot spot may be produced. Weight losses greater than 10% in rice stored only 1 year have been attributed to insect pests. In addition, the mere presence of live insects results in a special grade designation lowering market value.
Good housekeeping is recommended as the best method of eliminating or reducing insect infestation in any bin, elevator or warehouse where rough rice is stored. When emptied, a bin should be cleaned and sprayed with a residual spray approved for this purpose. Spaces under the floors of bins should be cleaned out and sprayed with a residual spray. In an elevator or warehouse, all junk, unused machinery, sacking, tools, and parts should be removed or stored in a compartment where dust and waste grain cannot accumulate. Waste grain, chaff, and dust should be removed from tunnels and lower areas in an elevator. Elevator boots, legs and heads, screw conveyor troughs, and spouting should be cleaned. Dust and waste grain should be removed from beams, ledges, and other places in the superstructure of storage buildings. All waste grain and dust gathered during cleaning operations should be promptly removed and disposed of. Mowing or complete removal of weeds and grass in areas surrounding the rice storage structure is recommended.
Aeration is a useful tool for reducing insect infestation. Insect activity is greatly inhibited at temperatures below 15.5°C. By scheduling aeration during periods of cold weather, rice can be maintained at a temperature below 15.5°C. during late fall and winter months.
Sprays for protecting rough rice against insect infestation are partially effective. These are applied while a bin is being filled as ready-to-use formulations or as concentrates to be mixed with water. Both premium grade Malathion and a mixture of pyrethrum plus piperonyl butoxide have been approved as protectants on rough rice. These materials are also used as surface sprays to combat such species as the Indian meal moth and the almond moth.
Whenever live insects or other evidence of active infestation are found in a rice bin, this bin should be fumigated to check the infestation and prevent it from spreading into other bins. Several materials are satisfactory for fumigating rough rice in bulk storage bins.
Aluminum phosphide is one of the most commonly used fumigants at the present time. It is a solid material formulated as both tablets and pellets. On exposure to atmospheric moisture the tablets react to produce phosphine gas (PH3), which affects neither germination nor milling quality; after the rice is milled, no undesirable residue remains. These tablets can be probed into a bin of rice, but the easiest method is to feed them into the grain stream as a bin is filled. Dosages should conform to recommendations on the manufacturer's label.
When bins are equipped for aeration, fumigants such as methyl bromide can be introduced into the air stream and distributed through the bulk of the rough rice. With this method, smaller dosages are relatively effective because of uniform distribution. It is especially useful in flat storages where routine turning of the rice is not practical.
To achieve uniform distribution of the fumigant, a return duct is installed between the overhead space in a bin and the fan to form a closed system. Within the closed system the air and fumigant mixture is recirculated as many times as is necessary to achieve an adequate concentration of the fumigant in all parts of the rice mass. Generally this is accomplished by operating the aeration system long enough to change the volume of air in the storage about five times. The volume of air in a rice bin may be estimated by assuming that half the space occupied by rough rice is air space and deducting half the volume of space occupied by rice from the internal volume of the structure. The airflow rate for fumigation is not critical. Good results have been obtained with any airflow rate that is adequate for cooling.
The direction of airflow may be either upwards or downwards through the rice. If the air moves downward, the fumigant will be released in head space above the rice. When the air direction is upward, the fumigant should be released into the air stream at the bottom of a bin.
A tight storage structure is needed for economical dosage. Cracks around doors, windows, or at the roofline should be sealed with masking tape. A dosage rate of 2 Ib. methyl bromide per 1,000 cu. ft. of bin space was found to be adequate at 70°F. (21°C.) or above. After adequate distribution of the fumigant, the fan is turned off. The air-fumigant mixture is allowed to settle for at least 24 hr., then the return duct is removed and the fan is used to expel the fumigant.
A serious limitation to the use of methyl bromide is that residues are cumulative with repeated treatment. The allowable tolerance (50 p.p.m.) may be exceeded with more than two treatments.
B. Other Pests
Two kinds of rats and house mice, Mus musculus, are the principal rodents that might be encountered in a rice ware-house. The rats are distinguishable by their method of entry. They are the burrowing rat, Rattus norvegicus, and the roof rat, Rattus rattus. A well designed warehouse can be built to exclude rodents, but numerous places of entry are available in most storage facilities.
An additional amount of grain is wasted due to rodent contamination. Other losses include damage to buildings, machinery, and electrical insulation from gnawing, and the cost of sickness due to rodent-borne diseases.
Complete exclusion is the best method of rodent control, but is not always possible, especially if a high population exists in adjacent areas. Good housekeeping, as recommended for insect control, is helpful. In addition, any nearby grain-refuse piles, trash dumps, or lumber and wood piles should be removed. A poisoning and trapping program may be necessary to eliminate the rodents that have found their way into a rice warehouse.
Bird contamination is as objectionable as insect or rodent contamination. Birds can be excluded by covering windows and other opening with hardware cloth. Cleaning up any spilled rice will make the area less attractive as a feeding ground for birds.
Wheat
I. ORIGIN OF WHEAT
The origin of the wheat plant is not known with certainty, although a good deal of evidence indicates that cultivated einkorn was developed from a type of wild grass native to the arid lands of Asia Minor. Emmer is generally regarded as one of the ancestors of the wheats common today, because it closely resembles the wild species of wheat found in the mountainous regions of Syria and Palestine.
Authorities do not agree on the exact place and time of the origin and earliest cultivation of wheat. The proportion of wheat to total grain used for food, was considerably larger than indicated by production, since barley and rye were used as feed, but scarcely any wheat was being fed. Likewise, wheat dominated domestic and international trade almost to the exclusion of all other cereals.
Nations with an adequate supply of wheat have, for centuries, been considered nutritionally well off.
Much research is in progress internationally to improve wheat production and quality. High-yielding dwarf wheats have resulted from crosses of Japanese with American varieties, and this has been the basis for increased wheat production in Northwestern U.S. in recent years. Dwarf wheats have been successful in increasing production in Mexico, India, and Pakistan.
Research efforts are being made to produce wheat varieties with higher protein content and with increased amounts of the essential amino acids. Work is being done to relate specific genes to the functional properties of wheat for bread-baking.
II. CLASSIFICATION OF WHEAT
Wheat can be classified in several ways, but the most fundamental distinction is based on the botany of the wheat plant.
vulgare. Common wheat durum, Durum wheat compactum. Club wheat turgidum, Poulard wheat dicoccum, Emmer spelta, Spelt polonicum. Polish wheat monococcum, Einkorn
For commercial purposes, the common wheats must be classified by other characteristics such as hard or soft, either red or white, and spring or winter habit. In addition, durum wheat is considered separately in some areas but not in others have established grain standards for wheat based on such factors as texture (hardness), color, foreign material, broken kernels, moisture, and test weight. Grain standards facilitate future trading, protect contracts, and obviate preserving the identity of each parcel of grain. Wheat is appraised throughout the world on the basis of the same general charachteristics, regardless of whether or not these factors are incorporated in written statements. Differences in varieties, environment, soil, and many other conditions all influence the composition, quality, and characteristics of wheat. It is not easy to provide a wheat classification that is satisfactory to producers, merchants, and processors alike but much progress has been made in this endeavor.
III. MOISTURE CONSIDERATION
Moisture content seldom is given sufficient consideration in the comparison of food commodities. An example concerns the production of potatoes which, when reported in bushels is larger than for any cereal grain; but the moisture content of potatoes averages approximately 78.8% whereas wheat has an average moisture content of about 12%. The world's 5,586.8 million cwt. of potatoes would contain 1,083.4 million cwt. of dry matter, whereas 305,100 thousand metric tons of wheat contains 5,929 million cwt. of dry matter, or about six times as much Also to be considered is that in the preparation of potatoes for use, often as much as one-fourth is removed in peeling and little or no food or fuel use is made of this material, whereas the one-fourth of the wheat kernel removed in processing during milling is utilized fully as animal feed.
Thus from the standpoints of production, dry-matter content, and low processing losses, wheat has advantages over most other agricultural products used for human food. Strangely, though, the per-capita consumption of barley, oats, and rice has remained about the same or has decreased only slightly in North America during the past 20 years, during which period the per-capita consumption of corn, rye, and wheat has declined considerably.
IV. COMPARISON OF NUTRIENT VALUES
The chemical composition of cereal grains varies widely, depending on environment, soil, and variety. The variations in protein, carbohydrates, lipids, mineral matter, and vitamins are so great that only an average proximate analysis can be given, as in Table 1. Although cereals are regarded primarily as energy foods, they are important sources of protein, and protein is one of the most difficult to obtain, and most expen-sive, essentials in a diet. It has long been recognized that the human body requires protein in sufficient amount and of the necessary amino acid composition. Although the quantity of protein in wheat can vary from 7 to 22%, the average value is higher than for other cereal grains, as shown in Figure 1. Not only is wheat a good source of protein, but as shown in Table 2, it also is comparable to other grains in distribution of essential amino acids. Cereal proteins, in general, do not rate high in biological value and require supplementation to sustain animal life.
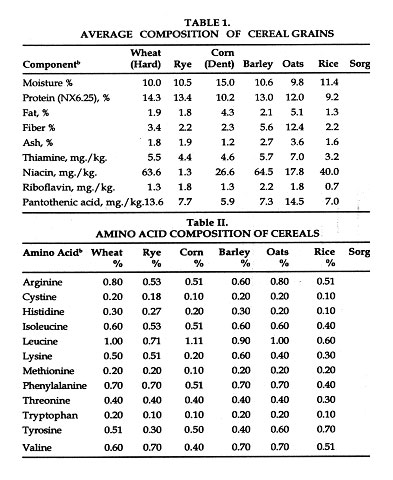
In lipid and mineral content the various major cereals vary considerably (Table 1), and wheat has no particular advant-age or disadvantage in respect to these constituents. The principal location of the lipids is the germ; the outer husk

Figure 1. Average protein content of major cereal grains. Cross-hatched section indicates content of essential amino acids.(and particularly the aleurone) holds the mineral matter. These parts are largely removed during processing. The quantities of lipids, minerals, and other constituents present in the whole grain are not necessarily related to their distribution in the processed food.
The carbohydrates from cereals are essentially equal nutritionally. Of the edible portion of cereals in human nutrition, approximately 70% consists of carbohydrates which are readily dIgestible. Likewise, in the vitamin content of cereals there are appreciable differences, but processing so changes and reduces the content in the edible portion that the values for the whole grain are not a guide to nutritional value in the diet.
V. WHEAT PRODUCTION
A. The Concept of Wheat Quality
There is considerable misconception regarding the quality of wheat produced in various locations, because most often when wheat quality is reported, the evaluation is based on shipments that have entered international trade. The appraisal of exports provides an important comparative quality evaluation of the wheat produced in various countries, but it is misleading to make the assumption, as is done in many reports, that exports are representative of the quality of the total wheat production. Apparently, no attempt has been made as yet to obtain an over-all appraisal of wheat quality throughout the major production areas. There are probably two main reasons why a study of this kind has not been undertaken: first, the principal interest in wheat quality is in that which enters international trade, and hence there is no great interest in quality as a statistic; and second, access to two of the largest wheat-producing areas.
b. Classes of Wheat
Although there are many ways in which wheat types could be differentiated, one of the most common is the division into Spring and Winter classes. Winter wheat is planted in late summer or fall to germinate and develop a young plant. The plant then lies dormant during the winter and growth is resumed in early spring. Winter wheat production, which has the advantage of utilizing fall moisture for germination and making effective use of sunshine, warmth, and rainfall from early spring, is predominant throughout the world where the soil does not freeze sufficiently to winter-kill the wheat plant.
Spring wheat, as the name implies, is planted in the spring and matures in late summer. Because the hazards are greater for obtaining a satisfactory harvest of spring wheat and yields are generally lower, there has been a gradual reduction in spring wheat production throughout the world.
VI. WHEAT USES
A. Food as the Main Use
Wheat, through the centuries, has been intimately associated with food uses for man. For a long, long time, man survived almost entirely on a meat diet which was supplied by hunting. Gradually, however, Homo sapiens learned to use his ingenuity, develop tools, make use of fire, and react to the influence of climatic change by adjustment in his habits rather than by passive acceptance of what nature supplied. A need arose for establishing more or less permanent settlements to supplant the temporary abodes used in the roaming life in the search for food. Man became a food gatherer in addition to a hunter. All classes of plant and animal life, including the seeds of the wild grasses, became sources of food.
Among the difficult problems to be solved in the use of grasses for food was that of securing a sufficient quantity of them in one place to justify the effort needed to isolate the edible inner portions of the kernels from the harsh and tasteless branny outer coat. Finding the solution to the problems of cultivating cereal grains, developing even crude methods for grinding, and processing the ground meal into useful foods have led to what is called civilization, or the advancement of the pattern of human life.
The history of the development of grinding implements, and the part these advances have had in the progress of civilization, have been interestingly told many times. Grains, in the Stone Age, were crushed between flat stones, the crushed material afterwards being moistened with water and made into a flat cake which was dried in the sun. At some later period, the practice of placing the cakes on hot stones or covering them with hot ashes was developed. Early efforts in the art of baking involved only meal, water, and heat, which produced bread made without fermentation, which we designated as unleavened. Such breads are common today in the oatcakes of Scotland, the Passover cakes of the Israelites, and the chapatties of India and Pakistan.
Although wheat is thought of first as a cereal, easily converted into human food, it has extensive feed and industrial uses also. Feed use has declined because, with wheat maintained throughout the world at a price level considerably above that of other grains, high price has discouraged its expanded use as feed for animals and poultry.
Recent emphasis on protein use has been stimulated by impact grinding and air classification of flour. By this new milling technique, fractions can be produced that are high in protein or starch. These products can find special food and industrial uses. Wheat starch can be utilized in food and also industrialy as other cereal starches are. When suitably modified, wheat proteins have been used for the manufacture of malt, for coffee substitutes, and for industrial alcohol production.
B. Feed Uses for Wheat
The usual conversion of wheat to a food product results in some portion of the kernel becoming an animal feed.
The wheat milling industry produces bran, red dog, germ, and shorts and these secondary fractions amount to approximately 25% of the whole grain. Although pro-ducts from the milling of wheat are among the early ingre-dients used by the feed industry, neither full nutritive nor economic value has been recognized generally. Recently efforts have been intensified to promote the more extensive use of mill products as feed by providing better knowledge of the constituents, availability, standardization, and price.
A development associated closely to the use of wheat for feed is Triticale, a cross of wheat (Triticum) and rye (Secale) developed in the hope of combining yield, disease resistance, and quality of wheat and rye in a single cereal species. This potential has not been achieved, but much progress has been made and it appears that protein content can be comparable to that of hard spring wheat combined with a slightly more balanced amino acid composition. The protein efficiency ratio of triticale is improved compared to wheat, and this can be an important feed advantage.
C. Endeavors to Find Industrial Uses for Wheat
Because of the recurring surplus grain problem, great efforts are being made to develop new industrial uses for wheat. New knowledge about the properties of wheat is coming to light, and many industrial uses for the constituents of wheat are known; but the great difficulty is economic. However, there is no reason to doubt that popular new and improved foods made from wheat will be developed in the future. As was discussed previously, the principal use for wheat products has been, for centuries, a leavened type of bread. Although many important changes have occurred in breadmaking since the beginning of recorded history, in many areas of the world bread is still produced in a primitive manner. Moreover, it is usually in such areas that per-capita consumption is highest, probably because cereal foods have always been the least expensive means of supplying energy requirements in the diet. But price and availability are not the complete explanation. When the population can procure a wider variety of foods, other foods are purchased, and bread occupies a less important place in the diet. In addition, bread made under modern conditions is often subject to criticism because of lack of taste, flavor, and chewability. Much research is in progress to find ways to intensify the sensations and aromas associated with fresh bread which seem to possess a universally favorable appeal.
In the years ahead, a major development which will in-crease the food usage for wheat, either by improving present products or creating new ones, is sure to occur. Should this not come to pass, however, the tremendous increase in popula-tion will require wheat products for food in ever-increasing quantities. Past civilizations have depended on wheat products for development and survival, and wheat is quite certain to continue to be the cereal of greatest importance for processing as a human food.
Barley
GENETICS AND BREEDING
A. Inheritance and Heritability
Barley is a crop with worldwide distribution, and it is a preeminent plant for use in experimental genetic studies, It is a diploid (2n=2x = 14) with a small number of chromosomes, which are relatively large (6-8 mm). Barley has a high degree of self-fertilization, but is easily hybridized. Several generations can be grown in one year over a wide array of environmental conditions. Seed can be stored for long periods and numerous genetic stocks are available for physiological and morphological characteristics.
B. Biotechnology
The barley nuclear genome contains nearly 12 billion base pairs of DNA among its seven pairs of chromosomes. In spite of its complexity, a series of molecular markers have been identifi ed which span half its recombinational distance. Linkage maps consisting largely of DNA markers are being constructed in the United Kingdom, Germany, the United States, and Canada. The application of these maps ranges from identifying the location of genes which modify characters including disease resistance, grain protein content, enzymatic characters, and extract, to providing excellent markers for varietal identification.
The barley chloroplast genome is small (@134,000 base pairs of DNA). The undetermined structure of the mitochondrial genome is much larger than the chloroplast, and it exhibits more variability than the chloroplast among genotypes of barley.
Transformation, the direct insertion of specific cloned genes, is now being attempted in barley. It has been reported to be successful in rye, soyabeans, and maize. Published examples of stable transformants in barley are not yet available.
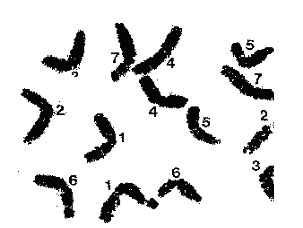
The molecular tools currently being developed have great promise to improve the efficiency with which we manipulate quality and other production-related quantitative characters and move the genes controlling their expression into varieties with better local or regional adaptation. Transformation has the potential to modify the resistance of barley to diseases such as Barley Yellow Dwarf Virus, to produce industrially proteins, and to modify the nutritional quality of the grain. Barley can be regenerated into fertile plants from various cells and tissues, but regeneration from protoplasts is not yet possible. These techniques would allow the use of embryo rescue, double haploid production, creating new variation, providing new cytoplasmic or chromosomal combinations, selection of mutants, in vitro fertilization, and attempting transformation.
E. Breeding
Barley is the most widely grown cereal crop, and with this diverse mix of environmental conditions the breeding objectives become quite diverse.
1. Population Breeding Methods
Barley was the first cereal crop used in an innovative way for the development of population breeding methods. Composite crosses were developed for many different specific uses by the scientists. Recurrent selection has been used in many crops, but a refinement was suggested "male sterile facilitated recurrent selection" (MSFRS). A disadvantage of the population method of breeding can be an inability to devise a suitable screening method on a single plant basis for the characteristics desired (examples are yield and quality).
2. Hybrid Barley
Methods of hybrid barley production have been reviewed recently. Methods proposed for the production of hybrid barley are use of synthetic hybrid populations, DDT resistance linked to male sterility, balanced tertiary trisomics, preflowering gene linked to male sterility, haplo-viables, chemical restoration of fertility, photoperiod sensitive male steriles, cytoplasmic male sterility, apomictic lines, and male gameticides. The most promising method appears to be the use of the cytoplasmic male sterility. Apparently sufficient heterosis exists in barley for it to be commercially successful, if a suitable method can be found. Other problems in hybrid barley production are sufficient female receptivity and male pollination ability. Composite crosses have been developed which greatly enhance outcrossing potential, but these genotypes need. to be integrated with appropriate parental stocks.
A. Plant
A covered barley kernel is shown germinating in Fig. 2 at from 3 to 7 days (depending on the cultivar) after conditions are favorable for germination. Fig. 3 shows a later stage in plant development, when tillering occurs. Barley has large overlapping auricles Fig. 4 compared to wheat (smaller nonoverlapping auricle) and oats (rudimentary auricles), which are readily distinguished at the seedling stage. The beginning of root development is also shown in Fig. 3.
Figure 5 shows root development in different types of barley depending on whether the area from which they come are dry (xerophytic) or wet (mesophytic). The apex of a barley root is shown in Fig. 6.
B. Spike
The spike is made up of spikelets with three florets attached to each of the nodes of the rachis. Two-rowed barleys have on one fertile floret, whereas six rowed barleys have all three fertile. Samples of two-and six-rowed barley kernels can be distinguished from each other because two thirds of the kernels in the six-rowed sample are twisted.
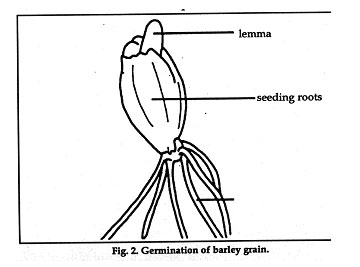
C. Kernel
The barley floret at anthesis is shown in Figure-7. Double fertilization of the barley egg cell is shown in Figure 8. A mature barley caryopsis or grain is shown in Figure-9. The kernel dry weight is made up of approximately the following percentages of tissue: husk and pericarp, 10%; aleurone and associated testa, pigment strands, and some nuclear tissue, 14%; starchy endosperm and nuclear remains in sheaf cells region, 73%; embryo, 3%.
A. Soil and Climatic Requirements
Barley grows best under cool dry conditions and can stand heat under low humidity or high humidity under cool conditions. It does not grow well under a hot humid environment primarily because of diseases. When both wheat and barley grow well in an area, wheat is the cereal of choice for food and barley for feed and malting. Barley matures more quickly than other cereals and thus can be grown at higher latitudes and altitudes. The winterhardiness of barley is not as good as that of rye or wheat, but is better than that of oats. Barley grows under a wide range of photoperiod, temperature, and moisture conditions, but it is grown best in the area where the cultivar was bred. Photoperiod was more important than

temperature in determining spike emergence in a longterm study, and available energy influenced the ripening phase more than temperature. Barley is the most drought-resistant cereal, but it may actually be more drought escaping due to the shorter maturation period.
Well-drained fertile loam or light clay soils are best for barley production, since sandy soils do not hold enough water and heavy clay soils may become waterlogged. Of the three cereals,barley, wheat, and oats, barley is more tolerant than the others of alkaline conditions and more sensitive to acid soils.
1. Rotations
The use of barley in a rotation depends on the length of the growing season, moisture available in the soil profile and expected rainfall, availability of other crops adapted to the region, disease problems, soil type, and soil fertility. Barley is a desirable companion crop, since its early maturity and early harvest aids in the establishment of the interseeded crop.
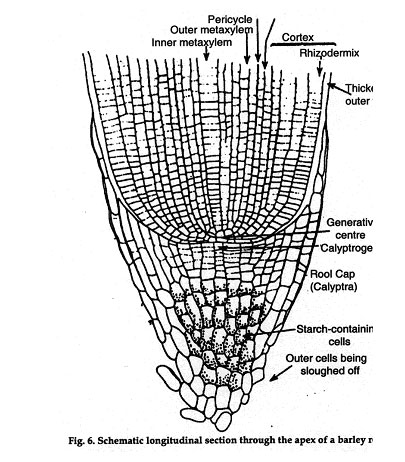
2. Planting
In general, spring barley should be seeded as early in the spring as possible for the highest yields, unless early spring frosts are a problem. Winter barley should not be planted too early in the fall, because of increased aphid and disease problems. In the southeast, barley is generally planted 1 or 2 weeks before winter wheat. Barley should be seeded 2-6 cm in depth and at a rate of 54-108 kg/ha.
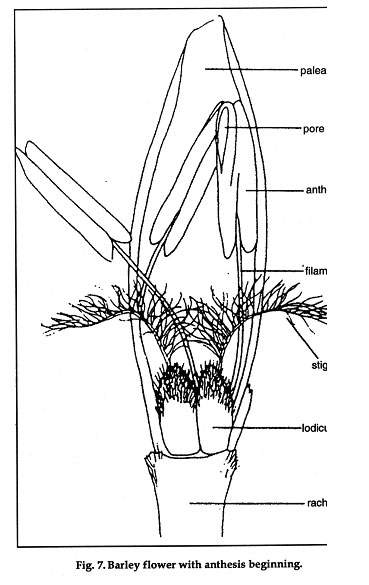
3. Fertilizing and Water Use
Barley responds well to applied fertilizers and increased water, but a good management plan must be in place to utilize these inputs. Soil tests are absolutely essential to apply the correct amount of fertilizer. Barley is not as lodging resistant as wheat and oats, so it may not tolerate such high fertility levels.
Nitrogen balance is the most critical nutritional factor determining the yield and quality of the barley crop and is especially critical when recropping after a nonlegume crop. Barley production is affected by the nitrogen source, type of growing season, the level of carryover in the soil, time and method of application, and cultivar grown. In subhumid and humid areas measurement of the residual nitrate, N, in the soil is not as effective in determining a correct recommendation as it is in the semi-arid areas. For winter, barley a split application of nitrogen in the fall and spring usually gives the best results. The interaction of nitrogen and water is the major factor determining yield. Much more N can be applied if' adequate water is available through irrigation with irrigation yield can be increased compared to dry land where yields stabilize at 112 kg/ha of N. The protein content of the grain Fig. 10 is also strongly influenced by N application. Excess N can decrease the value of malting barley, since high protein can result in rejection of the sample.
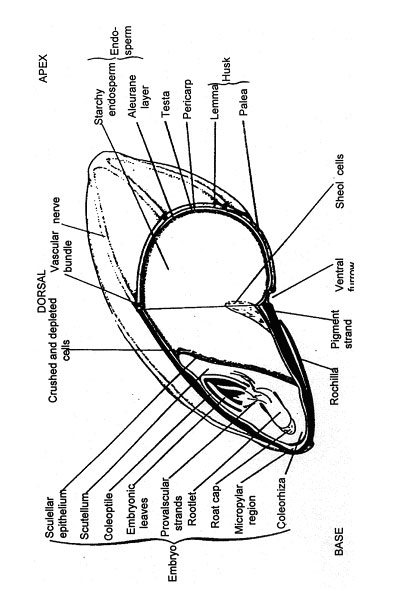
Correct timing of irrigation water is important for barley culture in maintenance of high yields and malting quality. If water is limited, the most critical time of application is the late boot-heading stage. This relationship between water and temperature is important in determining the time of application and amount of water to apply when irrigating barley.
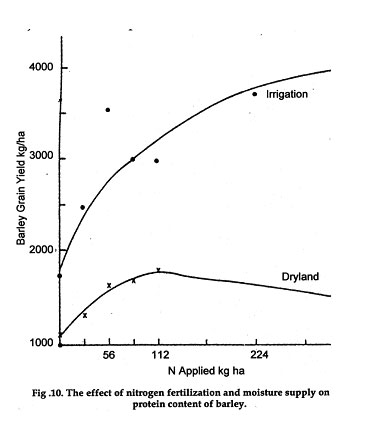
4. Harvesting
Barley harvested for grain can be cut and threshed directly with a combine at [14% moisture, but wheat and barley exhibit different threshing characteristics, so close attention is needed to cylinder speeds. Operators need to be especially careful with malting barley, because skinning and breaking of the kernels results in discounts or rejection by the maltster. Barley reaches physiological maturity at about 40% moisture and can be swathed at this moisture content or below with no loss of yield. Swathing can reduce kernel shattering, reduce losses for hail, insects, and frost and facilitate harvest of unevenly ripening fields.[/P]
C. Pest Control
I. Diseases
Although a strict definition of disease includes any abnormality of the plant, only infectious pathogens are considered in this section. Most barley diseases occur under irrigated or high-moisture conditions, but some diseases thrive when plants are stressed including those areas where disease has made barley unprofitable. North America is affected by about 15 economically important barley diseases. The region and climatic conditions of that area determine which ones are serious in a growing area. Diseases reduce quality as well as yield, causing shriveled kernels, high protein, reduced seed viability, and poor quality kernels. Disease organisms present may be toxic to animals and man. Diseases are controlled best by resistant cultivars, fungicides, or cultural practices. Breeding of disease-resistant cultivars is difficult since new pathogen strains often evolve as the new resistant cultivar is grown in monoculture. Attempts have been made to overcome some of the pathogen's ability to change through use of multilines or polygenic resistance.
2. Weeds
Weeds may be defined as "plants out of place." A wide spectrum of weeds are found in barley because it is grown in such a wide range of environments. Probably the most troubleÂsome weed in barley is wild oats (Avena fatua L.). Weeds compete for light, nutrients, and moisture and reduce yield, grain, and forage quality, increase labor and equipment costs, and reduce land values. Reduction in weeds can be accomplished through prevention, suppression, control, and eradication. Sanitation and prevention of weed establishment through cultural and chemical means is the best way of accomplishing the above objectives. Chemical control is only part of an integrated management program. Operators should use specific herbicides for specific weeds or herbicide combinations best suited to a particular situation. Effectiveness of the different chemicals vary, and tolerance of barley to the chemicals also should be taken into account. Barley cultivars also vary in sensitivity to a particular chemical.
3. Insects
Over 100 species of arthropod pests attack barley and they are estimated to cause 5-7% loss to the annual grain crop. Other losses are to the forage value of barley, reduction of quality of malting and food barley, and indirectly as vectors of virus diseases. The presence of insects does not automatically mean damage will occur, so an "economic injury level" must be reached before control is advisable. This injury level has been established for only a few pests of barley, and only a few pests such as the greenbug cause damage every year.
Crop damage due to insects usually occurs during the larval stage, with most insects having one generation per year (an exception is aphids, which have many generations per year). Some insects can be controlled with contact and some with systemic insecticides depending on their life cycle and method of plant infestation. Beneficial insects are an important agent in controlling many pest species, but they usually work slowly, and often chemical control is necessary to prevent crop loss. Care must be taken that the chemicals to control the pests do not also kill the predators and parasites.
V. CHEMICAL COMPOSITION
Barley grain is rich in starch and sugars, relatively poor in protein, and very low in fat. The husk (lemma and palea) is mostly composed· of lignin, pentosans, mannan, uronic acids, hemicelluloses, and cellulose fibers. Silica is present in the outer walls of the husk, and the awns contain large amounts of silica. The pericarp lacks lignin but otherwise resembles the husk in chemical composition. The testa contains crude cellulose and pigment strands of alkane waxes, which are a barrier for chemical substances and microbes. Polyphenols, which may complex with proteins, are abundant in the pericarp, testa, and aleurone layer. The aleurone has thick cell walls comprised of arabinoxylans and has aleurone grains of protein and phytic acid, spherosomes rich in fat, and abundant minerals. The subaleurone layer of the endosperm has relatively low starch content, is rich in proteins and b-amylase. The starchy endosperm is composed of about 85-89% starch enclosed in cell walls. b-Glucans make up 75% of the cell wall, and the rest is arabinoxylans. The embryo consists of about 7% cellulose, 14-17% lipids, 14-15% sucrose, 5-10% raffinose, 5-10% ash, and 34% protein. The cell walls of the embryo contains uronic acids, pectin, and hemicellulose (49).
A. Carbohydrates
1. Starch
Starch is a polysaccharide, a-glucan, and can be divided into straight-chain amylose and branched-chain amylopectin. The starch granules in the barley endosperm are laid down within amyloplasts and fall into two size groups: 1.7-2.5 mm and 22.5-47.5 mm. These starch grains contain traces of lipids, minerals, proteins, and nucleotides. The starch is synthesized in plastids, but the method is uncertain. The "diastatic" enzymes responsible for degrading the starch in germination are phosphorylase, a-glucosidase, a-amylase, b-amylase, debranching enzymes, and transglucosylase. The starch in the endosperm is 95% deposited in 11-28 days after ear emergence, and the ratio-of amylose to amylopec tin increases to the final ratio. In normal barleys the ratio of amylose to amylopectin is about 1 : 3, in high amylose Glacier about 1:1, and waxy barley is 97-100% amylopectin. Some workers have reported altered starch and free sugars in Riso 1508 barley, but others found no changes in the major carbohydrate constituents of the high-lysine barleys. The starch composition of several normal, high-lysine and high-sugar barleys are shown in Table 14 and vary from 21.2 to 64.4% in starch.
2. Soluble Sugars
At least nine monosaccharides and seven closely related compounds occur in barley. Glucose and fructose are found free and in combination, whereas other monosaccharides are polymerized as oligosaccharides, polysaccharides, glycosides, glycolipids, and glycoproteins. Soluble sugar content of normal barley is about 2-3%, hulless barleys 2-4%, high lysine barleys 2-6%, and high sugar barleys 7-13%. The total sugars and reducing sugars decline from anthesis to maturity, whereas nonreducing sugars are constant in amount during plant growth. The major sugar of living tissues of barley is sucrose.
3. Nonstarch Polysaccharides
These polysaccharides in barley are defined as "pectin fractions" or Hemicellulosic materials, which are then divided into gums if soluble in hot water and, hemicellulose if soluble in alkali. The material remaining after delignification and, removal of gums and hemicelluloses is termed "holocellulose." Endosperm gums consists of b-glucan and an arabinoxylan. Endosperm cell walls in barley are unique in cereals, since they completely enclose the cell-forming barriers to proteolytic and amylolytic enzymes. Some of the polysaccharides are linked to phenolic acid and some to lignin. The dietary fiber of barley is comprised of lignin plus the nonstarch poly-saccharides.
B. Protein
"Crude protein" (N x 6.25) contains about 80% "true protein" with the rest nonprotein N and amides (15). The growing plant contains 6-7%of the dry matter as protein, but this declines to 0.6-0.8% of the dry matter in latter growth stages. The limiting amino acid in barley protein is lysine followed by methionine, threonine, and tryptophane. When barley is grown at high levels of N fertilizer, the crude protein in the grain increases, but the proportion of lysine in the protein decline. The cereal proteins are classed as albumins, globulins, prolamines, and glutelins. In barley the albumins and globulins (salt soluble), which are rich in lysine (5-7%) and threonine, are mainly metabolic proteins. The prolamines are the major storage proteins of the endosperm and are low in lysine ([2%). The glutelins, also found in the endosperm, are associated with bound structural proteins of the membranes and are about 4% lysine. Hordeum spontaneum or wild barley lines were found to have protein from 15 to 28%, and lysine from 2.2 to 3.6%, indicating about 5-10% of the strains could be considered high-lysine, high-protein lines.[/P]
Fats
Barley lipid content is low (2-3%) compared to maize and oats. Triglycerides comprising 77.9% of barley lipids contain palmitic acid and the unsaturated fatty acids oleic, linoleic (principal one in barley kernel), and linolenic. The barley kernel also contains diglycerides, free sterols, free fatty acids, and sterol esters and hydrocarbons. Most of the lipids are in the endosperm (77%), embryo (18%), and hull (5%). The fat content of a developing plant increases and then may decline slightly. The fat content of normal covered, normal hulless, high-sugar, and high-lysine barleys range in percentage from 1.9 to 2.4, 2.7 to 3.9, 4.4 to 7.3, and 2.9 to 5.8, respectively.
D. Minerals
The ash content of barley (2-3%) is influenced by the growing season, soil zone, soil type, and soil fertility. The distribution of minerals is uneven throughout the kernel and the rachis (5-14%) and awns (17-38%) contain a large portion of the minerals. The ash content of barleys in range from 2.2 to 3.9%.
E. Vitamins
Barley is an excellent source of B-complex thiamine (B 1), pyridoxine (B6), riboflavin (B2), pantothenic acid and is also quite high in niacin, but only 10% of the niacin is available to monogastric animals. A small amount of vitamin E is found in the germ, and some biotin and folacin, but no carotene, or vitamin A, B 12, or D are found in ungerminated grain.
F. Phenolic Compounds
In plants the phenolic compounds can be divided into benzoic acids, cinnamic acids, terpenoids, and flavonoids. Barley contains a wide range of phenolic compounds in one form or another ranging from free and combined tyrosine, tyramine and derivatives, phenolic acids, esters and glycosides, and numerous other phenols through lignans and substances related to lignans. The anthocyanidines have been of interest recently since proanthocyanidin free barley used in brewing may prevent the formation of chill haze in beer.
VI. PROCESSING AND UTILIZATION
Barley is used worldwide for animal feed, brewing, and human food. In the United States the amount used for animal feed fluctuates with the supply available, since other feeds can be substituted if the supply is short and the price high. Malting barley demand is relatively inflexible from year to year since substitution by other crops is very limited.
A. Feed and Food Barley
1. Animal
a. Grain Barley nutritional quality refers to relative merit in providing essential total nutrients biologically balanced and available to humans and other animals. Payment to farmers is on the basis of quantity of feed barley produced, not feed quality produced, so breeders have concentrated on yield and malting quality. The best malting cultivars have the highest feed value. Barley is high in starch and sugars, low in fat, and relatively poor in protein.
The barley fiber is of little or no value for nonruminant animals, and the water-soluble mixed linked b-glucans may cause digestive problems in poultry. When barley grain is fed to swine, grinding, pelleting, cubing, rolling, and micronizing improves feed value. Pigs fed high-lysine barleys showed improved growth rate and increased biological value of the barley. Removal of hull genetically improved feed value of barley in most pig-feeding trials. Numerous reports show beneficial effects of feeding brewers dried grains to pigs, but the reasons for this improvement are not clear.
Barley fed to poultry in combination with corn improved egg production and feed efficiency compared to either fed alone. Although, barley has a higher protein content and a better balance of amino acids than corn, its commercial use in feeding poultry is restricted because of its lower productive energy and the sticky wet droppings that create sanitation problems. Supplementing barley with various enzyme preparations such as b-glucanase improved feed consumption, weight gain, feed efficiency, and cage cleanliness of chickens.
In the western, northwestern, and northern Great Plains of the United States, barley is a major energy source and major cereal grain of feedlots and dairy diets. When it is processed properly to obtain its maximum feeding value, it is equal to maize in feed value for ruminants (50). Cattle tend to bloat more and tire more quickly of barley than other cereal grains, but less roughage and protein supplementation is required because of the high-fiber and high-protein content of barley.
b. Hay, Silage, and Straw Barley hay should be cut when the nutritive value is suitable and yield is high. If harvested at the milk to soft dough stage and promptly dried, this objective can be reached. If awned barleys are harvested too late, the awns may damage animals' eyes, mouths, and gut linings.
If barley is ensiled it should contain more than 30% dry matter and enough carbohydrates to support adequate lactic fermentation (at early dough stage) Consistent differences have not been found in feed values of barley, wheat, and oat silage but all are inferior to maize silage for beef and dairy cattle. Barley straw is a useful roughage which can provide significant energy to ruminants. Cultivar differences in straw feeding value have been found. The barley straw has a low protein content, low digestibility, and low energy value, but chopping and grinding the straw increases its value. The digestibility of straw can be significantly increased by treatment with dilute solutions of NaOH. Proper treatment of straw with ammonia is more convenient than with NaOH and improves digestibility, energy value, protein, and inÂtake.
2. Human
In the last century barley has been used less for food than has wheat because of lesser palatability, baking quality, and milling characteristics. From the nutritional point of view, barley is equal to or better than wheat. Barley is eaten as popped barley, barley flakes, sprouts, barley starch and as sweeteners, barley malt flour supplements, malted milk, infant food syrups, barley tea or coffee substitute, and rice extender. After barley is steamed; milled, and supplemented with vitamins and minerals, it will produce an infant baby food where all the protein and carbohydrates come from barley. This infant food would be equal to presently produced commercial baby foods marketed in developing countries at a far lower cost. The b-glucan content of barley is of great interest to the chicken feed industry as well as from the human nutrition aspect. The b-glucans of barley will lower blood cholesterol levels in chickens, rats, and swine and in humans. Barley cultivars differ in b-glucan, content, which can be estimated by viscosity measurement. Soluble dietary fiber (relatively high in barley) may have a significant effect on human health and nutrition. Increased amounts of dietary fiber have been shown to help in controlling diabetes, hyperlipidemia, obesity, hypertension, coronary heart disease, and gastrointestinal disorders such as hiatus hernia, gallstones, appendicitis, diverticular disease of colon, bowel polyps and colorectal cancer, and hemorrhoids.
B. Malting Barley
1. Uses
Barley is used in preference to wheat and rye for malting because of the cemented lemma and palea, which protects the acrospire during germination, serves as an aid for filtering during brewing, and produces a firmer kernel at the high moisture content required in steeping and malting. Barley syrups and malt extracts, which are variable in composition are prepared by concentrating worts by evaporation.
C. Marketing
1. Classification and Prices Received
The classes and subclasses of barley are identified. Malting barley is usually purchased on the basis of samples drawn from rail cars loaded at country elevators and sold directly to the maltster as consigned by a commission firm, or sometimes directly to the maltster by farmer contract. Acceptable malting barley lots command a higher price than feed barley.
2. Storage
Most barley is harvested directly or from the swath by combining. Malting samples are binned separately by the farmer, country or regional elevator, and the maltster.
Current recommendations in local areas should be consulted for control of storage pests.