SOME NATURALLY OCCURRING BLACK PIGMENTS
Relatively few forms of life are completely devoid of black pigmentation. It varies in extent from mere specks and streaks to almost total coverage, and such specific names as niger, melanoxylon, etc., are common in biological nomenclature. Moreover many tissues blacken when subject to injury, disease or decay. Yet despite their considerable biological, aesthetic, and even political significance, the chemistry of black pigments remains obscure and we know comparatively little of their structure. This is in marked contrast to the status of other colouring matters but is hardly surprising as dark pigments are generally polymeric, irregular in structure, and likely to be conjugated with protein or carbohydrate. (If not polymeric, they usually prove to be dark red rather than black, e.g., the dihydroxyperylenequinone in the fungus Daldinia concentrica and the protoaphin present as its anion in the blood of black aphids.) Consequently the separation of colouring matter from extraneous material is often a major problem and the purified pigment is likely to be an intractable product with poor spectral characteristics. Structure determination by classical degradative methods is generally of limited value, and an indirect approach by examination of precursors is often more useful.
On the present evidence most, if not all, black pigments are formed in vivo by an oxidative process, the usual substrate being a phenol, more especially a catechol or 1,8-dihydroxynaphalene. Oxidation may lead directly to a quinone followed by oxidative polymerization, or may proceed by coupling of phenoxyl radicals. The formation of dark products by oxidation of simple quinones and quinols under both acid and alkaline conditions can readily be demonstrated in the laboratory. The nature of these polymeric products is indicated by the formation of di- and triphenyl derivatives, in addition to dark amorphous material, when simple benzoquinones undergo polymerization in acid solutions. More apposite is the recent observation that the enzymic oxidation of catechol affords a mixture of three tetrahydroxydiphenyls, e.g. (1), in addition to coloured materials. As the same products may be obtained by oxidation of catechol with silver nitrate or ferric chloride the reaction evidently proceeds by dimerization of mesomeric radicals, as indicated below. However, the conversion of catechol into o-benzoquinone by enzymic oxidation is also well established and if both compounds occur together the formation of dimers by an ionic mechanism cannot be excluded.
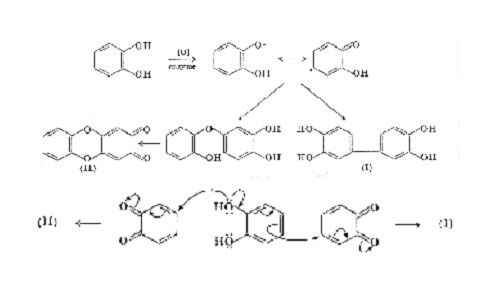
Further oxidation of catechol leads to the precipitation of a dark "catechol-melanin" most probably formed by a continuation of these
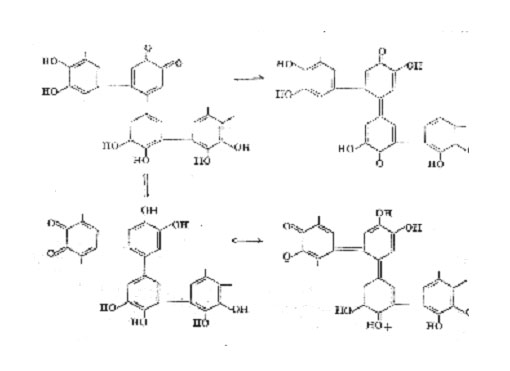
processes, together with additional oxidation of some of the benzenoid rings to quinonoid or semiquinonoid forms. The structures shown on p 100 are merely illustrative; all four unsubstituted positions in catechol are potential sites for oxidative couplings. In practice this scheme is probably complicated by the formation of ether linkages since the quinone (II) was found amongst the enzymic oxidation products of catechol, showing that carbon-oxygen coupling also takes place. The colour of an irregular polymer of the type indicated would be dependent upon the degree of oxidation and the coplanarity of consecutive rings, and would be modified in vivo by the introduction of auxochromic groups (e.g. by reaction with protein) and perhaps by association with metals. Numerous variations on this theme are possible and it seems likely that many natural dark pigments are formed by an oxidative process of this kind. Some examples are discussed in the sequel.
ANIMAL PIGMENTS
Melanins
The term melanin has been used to describe any natural dark-brown or black pigment irrespective of its structure or origin, and in much of the older literature the term has no chemical meaning. It is still loosely applied to any pigment of high molecular weight formed by the enzymic oxidation of phenols but this is a very wide definition which would include nearly every section of this review. Owing to the uncertainty regarding its structure it is desirable, nevertheless, to describe a melanin in terms of its origin and the definition now generally accepted is that melanins are pigments formed by the tyrosinase oxidation of tyrosine (III) or such closely related compounds as b-(3,4dihydroxyphenyl)-alanine, tyramine and 3-hydroxytyramine. Melanin can be obtained in vitro either by enzymic oxidation of tyrosine, etc., or by autoxidation, although the products obtained probably differ in structure. Melanins contain approximately 9% nitrogen and purified material can thereby be distinguished (at present) from other natural black polymers which contain little or no nitrogen.
Melanins occur almost throughout the phylogenetic scale but there is much uncertainty about many so-called melanins in plants and primitive animals. Very little work has been done on the plant pigments but chordate melanins, which play an important part in adaptive colouration and as protective agents against short-wave radiation, and more especially, mammalian melanins which are present in human skin and hair, and in certain malignant tumours, have been intensively studied. As the subject has been frequently reviewed, the present discussion is limited to recent work bearing on the structure of tyrosinemelanin.
The initial stages of melanogenesis up to the formation of 5,6-dihydroxyindole (IV) were first propounded by Raper' and are generally accepted.
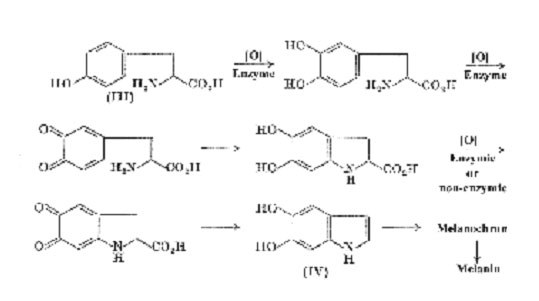
As the dihydroxyindole is a catechol, subsequent oxidation may proceed according to the schemes outlined on p. 100 but there are further possibilities in this case. It has been shown that nucleophilic addition of indoles to quinones occurs very readily to form dark blueviolet indolylquinones (e.g. (V)), and it might be expected that 5,6dihydroxyindole would be oxidized in vivo to the corresponding quinone as are the intermediate catechols which precede it in the biogenetic pathway. It is therefore possible for indole-5,6-quinone, if formed, to react with 5,6-dihydroxyindole (to form products analogous to (V)) or with itself (see below).
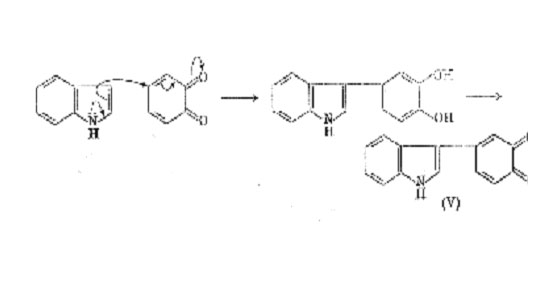
In a careful spectroscopic study of the tyrosinase oxidation of 5,6-di-hydroxyindole Bu'Lock has been able to detect what appears to be the initial dimeric stage (a tetrahydroxydi-indolyl) by its characteristic fluorescence. Immediate oxidation leads to a transient purple pigment (max. 530 mm), probably a 5,6 -dihydroxyindolylindole-5,6-quinone, which by further oxidative polymerization passes gradually through a purple phase with broader maxima around 540 mm, and finally into black melanin. The purple pigments (melanochrome) may be a mixture of low polymers intermediate between the dimer and the final product. Indole-5,6-quinone has never been isolated but 1methylindole-5,6-quinone (VII) can be obtained in solution by acid-catalysed dehydration of adrenochrome(VI). If this is done in the presence of 1,4-naphthaquinone a blue-green quinone, probably (VIII), is formed; there is also evidence that the same quinone (VII) can react with indoles. Hence, it is not surprising to find that 1-methylindole-5,6-quinone, which has both nucleophilic and electrophilic activity, polymerizes rapidly when generated in acid solution. However, this is a non-oxidative process
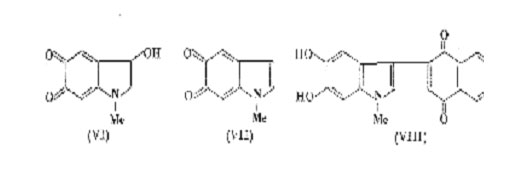
whereas, in normal melanogenesis, indole5,6-quinone would be formed by oxidation of 5,6-dihydroxyindole. The problem therefore is to determine whether 5,6-dihydroxyindole undergoes oxidative polymerization in catechol fashion, or by heterolytic indole/quinone addition, or, after oxidation to the quinone, in the manner of the I -methyl homologue, the nucleophilic centre in the pyrrole ring of one molecule reacting with the electrophilic centre in the quinone ring of another molecule.
Closely connected with the mechanistic problem is the uncertainty regarding the reactive centres in the polymerization process. On the basis of
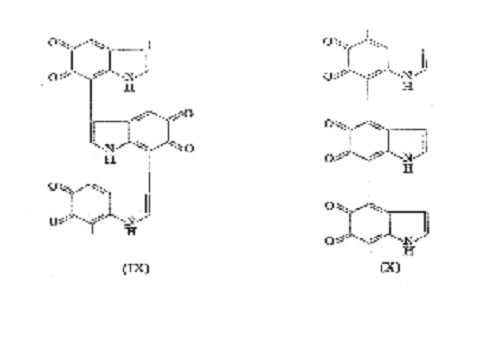
their model experiments with simple quinones and indoles. Harley-Mason suggested, some years ago, that melanin was formed by self-combination of indole-5,6-quinone at positions 3 and 7 to give a " back-bone " structure (IX). (The alternative 3-4 linkage was ruled out on steric grounds.) In addition, occasional cross-linkage at positions 2-4 or 2-7 was envisaged, resulting in a highly irregular three-dimensional polymer. This hypothesis was supported later by a comparative study of the rate of oxidation and the degree of polymerization obtained on autoxidation of a series of 5,6-dihydroxy indoles in which different nuclear positions were blocked by methyl groups but the results are not clear-cut. During the last decade Nicolaus have achieved some success by degradation of melanins with alkaline hydrogen peroxide. Treatment of synthetic tyrosine-melanin in this way gave very small yields of the pyrrolic acids (XI) and (XII) which were detected on paper chromatograms. These, and other acids, were also derived from natural melanins but it is noteworthy that in no case could pyrrole-2,3,4-tricarboxylic acid (XIII) be detected. If structure (IX) is correct, the acid (XIII) should appear on oxidative degradation. These results, which have been confirmed by Binns, imply that the 3-7 linked structure does not
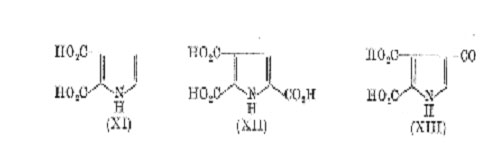
form a significant proportion of the melanin polymer. Admittedly the yields are minute, but the method must be considered reliable as pyrrole derivatives of known structure invariably yield the expected acids, and it is of particular importance that the acid (XIII) can be detected amongst the degradation products of violacein (XIV) when this is oxidized with alkaline hydrogen peroxide under the same conditions.18 Accordingly Mason, have proposed that the main back-
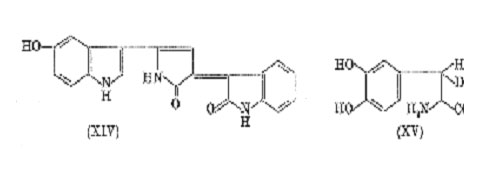
bone structure of melanin is a 4-7 linked structure (X) cross-linked at position 2.
Further support for this structure comes from the work of Swan using b-(3,4-dihydroxyphenyl) alanine monodeuterated on the b-carbon atom (XV). Oxidation of this compound either with molecular oxygen and mushroom tyrosinase at pH 6.8, or in the absence of enzyme at pH 8 (autoxidation), gave a melanin in which most of the deuterium was retained. Had oxidative condensation occurred at position 3 (indole numbering) the deuterium would have been lost. The work of Nicolaus, and of Swan, therefore suggests that all melanins are " catecholmelanins", the pyrrole rings providing subsidiary cross-linkages which add to the complexity and irregularity of the final product.
Most of these investigations have been carried out with model compounds and synthetic products but there is reasonably strong presumptive evidence that natural melanins (which are actually melano-proteins), at least mammalian melanin and probably Sepia melanin (from the ink of the cuttle fish), have the same general structure. Electron spin resonance spectra show that both natural and synthetic melanins have free radical character which is attributed to semiquinonoid structures. The polymers are therefore in a lower state of oxidation than that indicated by structures (IX) and (X).
Sclerotization
Another type of dark pigmentation in animals is that which usually accompanies the hardening of insect cuticle. In adult insects the cuticle is initially soft and colourless but in a few hours it becomes hard and brittle, and frequently darkens. The chemistry of the sclerotization process, which is of considerable importance in insect biology, is understood in general terms but there is considerable uncertainty and speculation about the details. As the present position has been surveyed very recently a brief summary of current views will suffice here. Several catechols have been detected in insect cuticle (probably originating from tyrosine in the blood) together with a polyphenoloxidase : it is therefore considered that oxidation to o-quinones takes place and these in turn react with amino groups in the cuticular protein, thereby effecting cross-linking between the polypeptide chains.
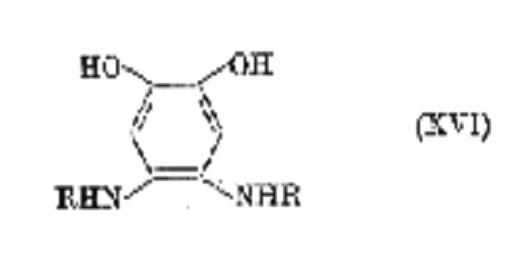
The process is analogous to the tanning of collagen by p-benzoquinone by which leather can be -produced. The cross-linked material is represented by (XVI; R and R' denote protein chains) in a reduced, colourless state, but the actual colour of the "tanned protein" (sclerotin) would again depend upon the degree of oxidation and steric factors. It is likely also that more complex cross-linking occurs involving the quinone carbonyl groups. Although hardening of insect cuticle can take place without darkening, fully sclerotized cuticle is usually dark brown or black. It is extremely difficult, however, to distinguish between the latter and melanoprotein, or catechol-melanins, which may also be present.
PLANT PIGMENTS
In plants the blackening of specific areas may occur during normal development, or following injury when tissues are broken and exposed to air, or as a post-mortem effect. The formation of dark pigments may also accompany manufacturing processes as, for example, in the manufacture of black tea and the extraction of liquorice. Much of this may be ascribed to phenol oxidation and undoubtedly where tyrosine, etc. and tyrosinase are both present (e.g. in the banana), melanin formation takes place, but there are also black pigments of non-phenolic origin. Very little is known of the chemistry of black pigmentation in plants, although for centuries the formation of black pigments by phenol oxidation has been exploited in China and Japan for the lacquering of furniture. The natural varnish is a greyish-white exudate obtained from various Anacardiaceae (chiefly Rhus spp.) which is rapidly oxidized by air in the presence of laccase, the substrate being a mixture of alkylcatechols (urushiol and related compounds). Quinols are also implicated in the formation of black pigments. Arbutin, the monoglucoside of quinol, occurs in many plants, and it has been suggested that in some cases the blackening of senescent leaves (e.g., Orobus niger L., Pyrus communis L.) is caused by oxidation of the aglycone after enzymic hydrolysis.
Numerous dark pigments originate from glucosides. According to Bridel; a glucoside of unknown structure, monotropein, is responsible for the blackening of Monotropa hypopitys Walt. This compound may be related to aucubin, the probable cause of blackening in dried Melampyrum spp. Several glucosides of this type have been isolated from the leaves, bark or fruits, of many plants. These enolic hemi-acetal glucosides, represented by plumieride (XVII). (from Plumiera acutifolia Poir.) and aucubin (XVIII) (distributed in at least eight families), are characterized by the rapid formation of amorphous, blackish-brown, polymeric products on hydrolysis. It is now recognized that bakankosin (Strychnos spp.), verbenalin (Verbena spp., Cornus florida L. (Cornaceae)), genipin (Genipa americana L. (Rubiaceae)) and asperuloside (Rubiaceae) belong to this group, which may be extensive. Asperuloside has been detected in over seventy Coprosma spp., the green leaves of which blacken when bruised, due to enzymic hydrolysis of the glucoside and subsequent reaction of the aglycone. All these glucosides yield dialdehydes on hydrolysis but nothing is known of the structure of the dark polymeric materials to which
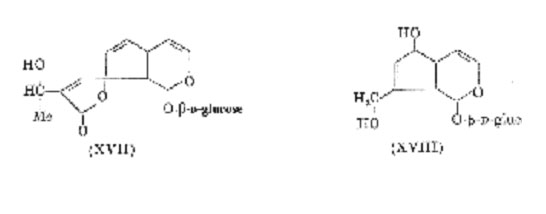
they give rise. The juice of the ripe fruits of G. americana has been used as a black cosmetic, and there are indications that the indelible bluish-violet colour which is rapidly produced when genipin is brought into contact with the skin, is formed by reaction with amino-acids.
Humic acids
By definition humic acids are dark amorphous materials occurring in soil, peat and low-rank coal, which are soluble in alkali and precipitated by acids. Were it not for the restrictive clause denoting their origin, this definition would probably include most naturally occurring dark pigments. Consequently it has little chemical meaning, and in any case it is quite arbitrary since much of the alkali-soluble material extracted from soil which is not precipitated by acid (called fulvic acid), is not significantly different in chemical nature from that which is.
Little that is useful can be said concerning the structure of these complex products. Current views, indicating a general agreement to differ, are set forth in the proceedings of a recent symposium. Undoubtedly the apparent lack of agreement amongst workers in this field stems, in part, from variations in the humic acids themselves, consequent upon differences in the plant remains from which they originate, the soil, climate , microflora, drainage, etc. Humic acids incorporate caxbohydrate and amino-acid components which can be removed by hydrolytic procedures but the manner of their combination with the aromatic core of the complex is not known. The nitrogen content can usually be reduced to c. 2% or less by suitable treatment, and some investigators regard humic acids as essentially nitrogen-free. Metals may also be present, notably chelated iron, which causes some further darkening of the organic material.
It is generally conceded that the core of humic acid consists of polymeric phenolic material. This may be derived from leaf polyphenols, by breakdown of lignin, by microbial biosynthesis from carbohydrates, or in other ways. It has been shown that alkaline degradation of certain humic acids affords phenolic fragments characteristic of lignin : recent examples include vanillin, p-hydroxybenzaldehyde and syringaldehyde derived from a peat humic acid by nitrobenzene oxidation,and a series of vanillin derivatives which were detectable on paper chromatograms after oxidation of a podzol humic acid with copper oxide. The relationship of peat humic acid to lignin is also supported by infra-red data.
Phenols are frequent metabolites of micro-organisms in pure culture, and although it is not yet established that this biosynthetic process contributes to humic acid formation in soil it is known that various fungi and bacteria can form products resembling humic acid. An example is the substance aspergillin, a metabolite of Aspergillus niger van Tiegh. When polysaccharide and protein have been removed, it is a jet black powder, free of nitrogen and iron. Like humic acids it contains carboxyl groups, and yields mellitic acid and oxalic acid on degradation. In general, aspergillin behaves like a cross-linked polymer closely resembling humic acid. The infra-red spectrum is not unlike that of a humic acid from a low-rank coal and its X-ray pattern is similar to that of humic acid from various sources.
The oxidative polymerization of simple catechols and quinols has already been discussed. A persistent theory of humic acid formation postulates that the aromatic core is formed by this process, together with additional oxidation giving rise to carboxyl groups. Numerous artificial humic acids have been prepared by oxidation of simple model compounds, and if this is done in the presence of ammonia or amino-acids it can be shown that some of the nitrogen is chemically bound to the polymer, presumably by addition to quinonoid structures. Synthetic "humic acids" show a general resemblance to the natural products but as the latter have few really characteristic features, such comparisons are not particularly convincing. A partially quinonoid structure could account for the dark colour of humic acids, and the reversible reduction which they undergo in alkaline solution (the colour becoming lighter), is also indicative of a quinonoid constitution, but there is little supporting evidence.
1,8-Dihydroxynaphthalene poIymers
About twenty-five compounds related to 1,8-dihydroxynaphthalene and 8-hydroxy-1,4-naphthaquinone have been isolated from fungi and flowering plants, and it is reasonably certain that all of these are biosynthesized from acetate units. The following is illustrative.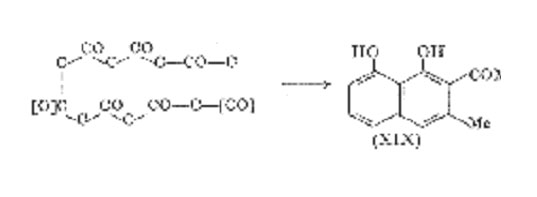
Compounds of the type (XIX) which lack a carbonyl group are very susceptible to oxidation and autoxidation, and in the natural products one of the hydroxyl groups is often methylated or combined with a sugar. Oxidation and autoxidation converts 1,8-dihydroxynaphthalene mainly into dark polymeric products (exceptionally, Fremy's salt affords 8-hydroxy-1,4-naphthaquinone in good yield), and this occurs by enzymic oxidation in the large Ascomycete, Daldinia concentrica (Bolt.) Ces. & de Not. In the wild state the fruit bodies of this fungus are pigmented by an almost black perylenequinone and a black polymer, both derived from 1,8-dihydroxynaphthalene. By studying laboratory cultures of various strains of D. concentrica which were subject to enzyme deficiencies, Allport were able to isolate a series of C10 (and C8) aromatic metabolites whose derivation from acetate was established. 1,8-Dihydroxynaphthalene itself was not detected, but there is no doubt that it is a metabolic intermediate since its mono- and dimethyl ethers could be isolated from strains deficient in oxidase, whereas the wild type formed the dinaphthyl (XXI), the quinone (XX), and insoluble black polymer (XXII) some of which
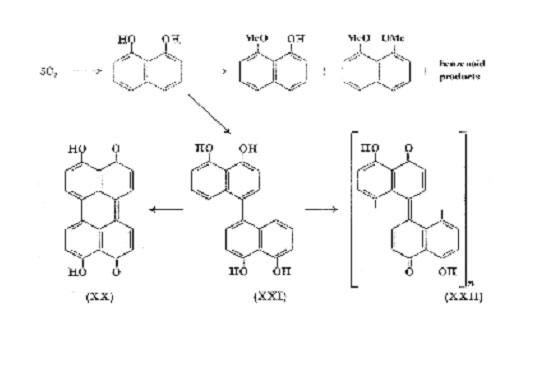
was firmly bound to the cell wall. Furthermore the dimeric and polymeric products can also be obtained in vitro by oxidation of 1,8-dihydroxynaphthalene with ferricyanide or with an aqueous extract of D. concentrica. The dehydrogenation of phenols in this manner is a common metabolic process, and it can be seen that the initial dimer (XXI) can form either the quinone (XX) by intramolecular oxidation, or the polymer (XXII) by intermolecular coupling. Extensive coplanar polymerization is improbable on steric grounds but the unit (XXII; n = 1 or 2) would be virtually black. Quinonoid properties are indicated by its reversible reduction, the leuco compound being a light tan colour.
It is unlikely that the Daldinia type of polymer is unique in Nature and further examples may be looked for amongst plants which produce similar naphthalene derivatives. Four substituted 1,8-dihydroxynaphthalenes (a- and b- sorinin, musizin (XIX), and 2-acetyl-1,8-dihydroxynaphthalene) have been found in Rhamnaceae which is not a family noted for dark pigmentation, but it may be significant that such compounds (and also perihydroxynaphtha-quinones) occur in several species of Diospyros (Ebenaceae) from which ebony is obtained. The leaves and fruits of many Diospyros spp. also blacken on drying. In particular Macassar ebony, the heartwood of D. celebica Bakh., contains macassar II (XXIII) and macassar III (probably the methyl ether of (XXIII)), and the dinaphthyl derivative, diospyrol (XXIV), has been isolated from the fruits of D. ehretioides Wall. The structure allotted to the latter may require modification but it is almost certainly a bis-1, 8-dihydroxynaphthalene and
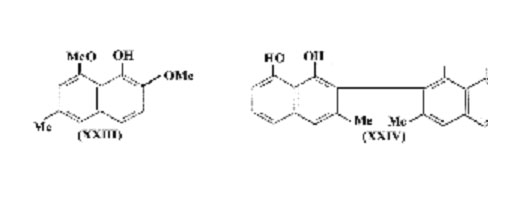
is very sensitive to oxidation. In many species of Diospyros the light sapwood rapidly turns black or dark brown on exposure to air and there is a tendency to develop a dark traumatic heartwood on injury. It seems a reasonable speculation that the dark pigments of ebony are, in part, polymers of the Daldinia type and experimental evidence is now being sought.
Previous attempts to elucidate the structure of the ebony pigments met with little success, a major difficulty being the separation of the polymeric colouring matter from lignin and cellulose. Wedekind55 heated "West African ebony" with dioxan-hydrochloric acid, followed by shaking with 72% sulphuric acid, and a final treatment with phenol. The residual black amorphous product was deemed to be the purified pigment. Analysis suggested a molecular formula (C3H2O)n (containing methoxyl groups), but degradative experiments afforded no recognizable products and the isolation procedure is open to criticism. Griffioen has obtained a brown amorphous substance from a Diospyros heartwood by alkaline extraction. On fusion with potash it afforded phloroglucinol, catechol and protocatechuic acid, and showed a general resemblance to humic acids. Both authors concluded that the dark pigments were derived from lignin.
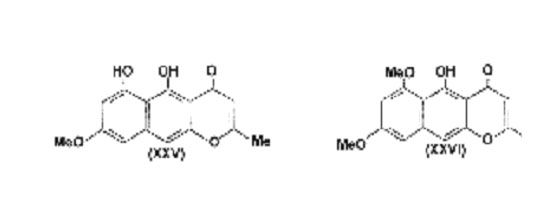
Finally it is not without interest that several strains of Aspergillus niger produce small amounts of "asperxanthone" (a derivative of rubro-fusarin), in addition to aspergillin. It is now known that rubrofusarin is not a xanthone but has structure (XXV), whence it follows that "asperxanthone" is (XXVI), related to 1,8 dihydroxynaphthalene.
Natural Dyes for Food
Natural Colourants
The trend towards natural ingredients in foodstuffs is continuing and this is evidenced by consumer acceptance of 'natural' foods and the various national regulations which completely or selectively ban artificial colours from food. Currently, the degree of safety testing required of a synthetic compound designed for food use is prohibitively expensive and the less stringent testing designated for natural compounds has obvious attractions. Whether the situation will become more or less restrictive is difficult to say. In the first volume of this series, Walford suggested that a world-wide list of permitted colours be established to ease the export of processed foods. This ideal has still to be achieved but the permitted lists of each country and the associated labelling regulations generally favour the use of natural colours. It should be emphasised that the current appeal of natural colourants is based solely on their consumer acceptance and the peculiar regulation that allows a natural colour to be listed with perfunctory testing while a pure, synthetic compound is subject to practically every toxicological test ever devised. A change in legislation could alter the situation dramatically as some of the natural colourants would undoubtedly fail the full toxicological testing. Despite the current acceptance of natural colours, their effect on human metabolism should not be ignored. Pharmacological properties have been ascribed to some of the phenolic natural colourants and toxicological data have also been published on some. While mutagenic properties have been ascribed to quercetin (when tested against Salmonella) the beneficial effects of flavonols and other polyphenolics have also been illustrated. Besides the pigments themselves, the effect of metabolites or degradation products of the natural colourants on the human system should also be considered.
Natural Colours Presently Used in Food
At present the use of natural colourants in food is limited, due to their instability, poor tinctorial power and the limited range of colours available.
Natural colourants produced for use in an analogous way to the coal-tar dyes are crude extracts of pigments which are basically unstable. The apparent stability of some food products owes more to the amount of pigment present than to the tinctorial power of the pigment itself. For example beetroot, even after prolonged cooking, retains an attractive deep red colour, but the extracted pigment is unstable. Anthocyanin preparations have found use in some products, but their colour variation with pH has restricted their use, mainly to acidic products. However, in nature, the flavonoids produce colours from white through, yellow, red and blue to black at the pH of cell sap. The potential colouring power of flavonoids is therefore great.
Carotenoids are relatively stable and there is sufficient demand to make complex chemical syntheses of 'nature-identical' carotenoids worthwhile. Their colour range is limited to yellow/orange/red and they are naturally oil soluble although water-soluble forms are available.
Chlorophylls are used as colourants in a range of foodstuffs and both natural chlorophyll (containing magnesium as the central metal ion) and 'copper chlorophylls' (copper substituted for magnesium) are available. Both chlorophyll and copper chlorophyll are manufactured in oil-soluble and water-soluble forms by selective retention or hydrolysis of the phytol side-chain.
Apart from these three plant pigments, there are others such as red beet extract (betanin), cochineal, turmeric extract, etc. which have found use in food.
Methods of Improving Natural Colourants
Granted that many of the natural pigments presently available are unsatisfactory for one reason or another, attempts have- been made to improve the situation by various means. One approach has been to search the plant, animal and microbial kingdoms for pigments which are stable under conditions prevailing in foods (pH 2-8, temperature - 20 to 120oC, presence of oxygen, sulphur dioxide etc.). Reports of colourants from novel sources are listed and discussed in Section 2. A second approach has been the prevention of colour loss from foods. After all, it is the destruction of the original colour that necessitates addition of extra colour and, if the loss can be kept to an acceptable level, colouring is unnecessary. Both chemical and physical methods have been used to prevent destruction of natural colours in foodstuffs. However, this applies only to foods which naturally contain colour. Some foods that are formulated from essentially colourless ingredients (e.g. biscuits) will still require colour addition.
The stability of some natural colourants in vivo has initiated a third approach. For instance, stable coloured complexes have been isolated from several sources (e.g. blueberries) and shown to consist of an intimate mixture of polysaccharide, anthocyanin and metal ions. In a similar way, chlorophyll degradation in whole tissue is slow when chlorophyll is in its natural state; bound to protein. Only above the denaturation temperature of the protein is chlorophyll breakdown substantially accelerated. A greater awareness of the form in which natural colourants exist in nature might lead to more stable forms. At present the pigments are available as crude extracts which produce coloration very different from that found in the original material. Thus black grape skins yield an anthocyanin which is bright red below pH 2 but colourless or yellow at grape pH. Few attempts have been made to upgrade the extracts apart from the addition of carriers to improve solubilisation and preservatives to stop oxidation or microbial infestation.
A different approach to the improvement of food colour has been taken by some plant breeders who have attempted to breed strains with increased pigmentation. The philosophy behind this is that the increased amount of pigment will allow an acceptable colour to be achieved even allowing for losses during processing. This approach lies outside the scope of this chapter but, for example, strawberries which have uniform colouration throughout the fruit have been bred and used in canned packs.
A common feature of all approaches is that, to remain natural, a natural colourant must be stabilised in a natural way. The legislative definition of 'natural way' has not yet been tested but it would seem that treatments such as heating, extraction with permitted solvents, reaction with enzymes or permitted food additives would be within the spirit of the 'natural' definition. Apart from the legal shackles which hinder the chemist's search for an acceptable stable natural colourant, the cost of the finished product must also be considered. Unless an expensive process confers a vast benefit on the product, it must be considered uneconomic. The economic effects of using natural colourants have been calculated by McClelland who derived a Cost in Use Disadvantage (CIUD) index to compare the costs of natural and synthetic colourants. Natural colourants were significantly more costly than the synthetics but, in McClelland's view, the increase was not prohibitive. Ultimately the consumer will settle the issue by buying or rejecting the more expensive naturally-coloured products.
In this context it is interesting to note the economics of biotechnological processes, as production of natural colours; by these means has been suggested. In the report Application of Biotechnology to the Food Industry-An Appraisal the authors state that '...the cost of raw material production based on biomass is greater than £2000 tonne-1 (1980 prices). For low volume metabolic products produced by plant cell culture, the critical cost is in the order of £ 100 000 tonne-1'. These latter costs may be acceptable to the pharmaceutical industry but they limit the production of natural food colourants by plant cell culture. Colourants presently available sell for £5000-£150000 tonne-1, depending largely on their tinctorial power, so production by microbial means may be feasible.
Thus the already onerous task of improving natural colourants is additionally complicated by the financial and legislative constraints. The following sections tackle the various approaches taken to improve natural colourants by discussing the merits and feasibility of each.
NOVEL SOURCES OF NATURAL COLOURANTS
In the search for a colourant that has the properties desired for food use practically every part of the biosphere has been investigated. The following sections describe recent discoveries cited in patents and journals and listed here in sections on microbial, animal and plant sources. In addition, some earlier work which falls outside the period of review but which is considered important has also been incorporated.
Microbial Sources
Production of materials by the culture of micro-organisms has several advantages. The rapid growth of microbes cuts production time to a matter of days and the process lends itself to continuous operation. Compared to plant or animal sources, the production is flexible and can easily be controlled. Microbes produce a variety of colourants such as chlorophyll and carotenoids as well as some unique pigments. By incorporating suitable genetic material into selected microbes, it may be possible in the
TABLE I
MICROBIAL SOURCES OF NATURAL COLOURANTS
Organism |
Description |
Monascus anka |
Production and extraction of red pigment |
Monascus anka |
Hyperpigment productive mutant |
Monascus anka |
Structure of ankaflavin |
Monascus purpureus |
Antibiotic production |
Monascas purpureus |
Incorporation into petfood |
Monascus spp. |
Wheat, starch, corn meal substrate |
Monascus spp. |
Combination with amino groups |
Monascus spp. |
Effect of combination on colour |
Monascus spp. |
Controlled culture gives major pigment |
Monascus spp. |
Water soluble pigment with protein |
Monascus spp. |
Yellow pigment from Monascus |
Monascus spp. |
Quantity and quality of pigments |
Monascus spp. |
Edible natural red dyestuff |
Monascus spp. |
Pigment in sausages |
Chlorella |
Red Carotenoid pigments |
Yeast |
Heated autolysate gives colour |
Blue-green alga |
Blue phycocyanin pigment |
Nocardia |
Red pigment colours fish paste |
future to produce other pigments not normally associated with micro-organisms, e.g. anthocyanins.
Most of the reports of food colourants from microbial sources involve Monascus species (Table 1). Traditionally, micro-organisms of this genus have been grown on rice until the whole mass becomes coloured by the red mycelia. The mass is then dried and powdered to produce a colourant long used in foods of oriental origin. Alternatively, the whole mass (called angkak by the Chinese) is consumed as food. The structures of the colourants have been reported and are shown in Fig. 1. In this form, the pigment is orange in colour but it readily reacts with molecules containing amino groups to form red compounds with the typical structure shown in Fig. 2.
A wide range of naturally occurring compounds containing amino groups have been suggested as substituents and they also confer a degree of water solubility on the molecule which, without substitution, is normally fat soluble. Thus Moll and Farr23 produced red colourants substituted with chitosan, ethanolamine or hexosamines. It was suggested that the use of chitosan as the adjunct was beneficial as chitosan is readily available from the insect kingdom and is not digested by humans which means it has a minimal effect on the human organism.
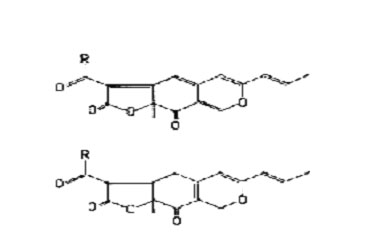
FIG. 1. Structure of pigments from Monascus (above) R = nC5H11, monascin;
R=nC7H15, ankaflavin (below) R=nC5H rubropunctatin; R=nC7H15, monascorubrin.
Instead of extracting the pigment from Monascus, some workers have added the whole coloured substrate to foods for colouring purposes. Corn or rice was used and incorporated into a pet food at a level of 1-5 % to impart a red colour. Successful semi-moist pet foods were also formulated using extracted pigments dissolved in various glycols.
Careful control of the culture conditions selectively improves the yield of the various pigments. Thus under appropriate conditions, the orange pigment can be produced as the major coloured pigment. The production of a single pigment instead of a mixture prevents colour variation between batches due to changes in pigment composition. Culture of Monascus on powdered bread is reported to produce yellow pigments while solid culture of a M. anka strain gave 'hyperpigment production'.
Other micro-organisms have been cultured and their pigments extracted for use as food colourants. An extract of Chlorella yielded 'pure carotene
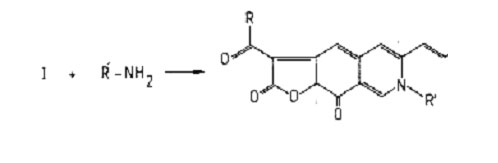
Fig. 2. Structure of substituted pigments from Monascus. R = aliphatic radical,
R´ = radical from R´ - NH2.
and a red pigment' claimed to be suitable for food use as was phycocyanin extracted from a blue-green alga and a red pigment from Nocardia.
A novel colour claimed to be suitable for food use was produced by heating a 10% yeast autolysate with sugar at 150oC, then adding 1% food grade soda and after 30-40 min adding 0.05% ammonium chloride. Even though the ingredients may have been of food grade, it seems unlikely that the method of production would be considered 'natural'.
Although cultivation of micro-organisms for the production of food colourants has attractions, these must be measured against the financial, legislative and user constraints. Table 2 summarises the costs of colourant production from Monascus under the headings raw material cost (based on laboratory SLR reagents and the quoted papers), processing cost and the cost of a tonne of pigment based on a 5% yield. No allowance has been made for extraction of the pigment and related solvent and solvent recovery processes.
Although these figures are extremely approximate due to the scarcity of information, they do show that production of Monascus pigment using a solid agar substrate is extremely expensive. The simpler substrates such as molasses or corn bring the production cost more into line with the approximate cost of the cheaper colourants whose current prices are given in Table 3. Without more details of the tinctorial power of Monascus pigments it is impossible to say whether the estimated prices in Table 2 would be acceptable.
The attitude of regulatory authorities to microbially produced colourants is impossible to anticipate, but no doubt the strains would have
TABLE 3
APPROXIMATE 1982 PRICES OF FOOD COLOURANTS
Colourant | Price £/tonne |
Yellow/orange azo dyes |
5000 |
Erythrosine |
25000 |
Brilliant Blue FCF |
15000 |
Cochineal |
150000 |
to be proved pathogen free and free of any toxic components. The precedent established by the FDA which limits the production of enzymes for food use to just three organisms (Aspergillus niger, Aspergillus oryzae and Bacillus subtilis) might count against Monascus produced pigments. Also the fact that Monascus purpureus has been reported to produce antibiotics simultaneously with pigments will require close attention.
There is still no substantial evidence to suggest that the pigments from Monascus are superior to other natural colourants. Potentially their orange or red colour is suitable for food use, their long usage in Oriental foods is also in their favour and the property of reacting with amino-containing compounds suggests that they could easily be incorporated into food systems. They appear to be stable in the pH range 2-10 although below pH 2 precipitation occurs on standing. Autoclaving of pigment-protein complexes resulted in cloudy solutions presumably due to precipitation of the protein, but methanolic extracts of the native pigments survived 3 h refluxing at 66oC.
When used in a sausage formulation, monascorubine was well absorbed by meat and non-meat proteins giving them a pink colour of cooked sausage. The pigment was stable to the heat treatment received during cooking and was not released into the meat juice nor did it colour the fat portion of the sausage.
Animal Sources
The most common animal pigments of use as food colourants are those based on the haem structure. In nature, haem is combined with proteins and occurs mainly as haemoglobin and myoglobin. Although the appearance of these two components is attractive when they are oxygenated (bright red) the colour produced on heating is typically brown (e.g. cooked meat) and removal of oxygen in the native state gives rise to the blue/purplish colour of venous blood. The colour changes are due to the
TABLE 4
NOVEL ANIMAL SOURCES OF NATURAL COLOURANTS
Description
Haem-imidazole compound preparation and stability
Modified preparation and stability
Haem pigments stabilised with carbon monoxide
Haem pigments stabilised with carbon monoxide (in a semi-moist petfood)
Cobalt complex of haematoporphyrin
Extraction of cobalt complex of haematoporphyrin
Haem and haem/nitrite pigment for fibre coloration
Nitrosylhaemoglobin in fibre spinning
S-nitrosocysteine colouration of haem pigments
Ferrihaemochrome in fish-sausage
Oil soluble pigment from Euphasia crustacean
oxidation state of the central iron atom in the haem portion of the molecule and the nature of the ligands surrounding the iron atom. The bright red colour of freshly cut meat or arterial blood is due to oxygen binding as a ligand to the iron atom which is in the ferrous state. However oxygen does not bind very strongly (it is easily given up to tissues from blood) and it is known that other ligands bind more strongly, stabilising the molecule and preserving the red colour. Thus, one method of producing stable colourants is to find a suitable ligand to stabilise the natural haem pigments. Cyanide is an excellent agent for this purpose but is obviously unsuitable for food use. Nitrite is also a ligand and it is presently used in food products although there is some controversy over its use as an additive.
Ligands suggested for stabilisation of haem pigments are imidazole (and its derivatives), S-nitrosocysteine, carbon monoxide and nitrite (Table 4). The imidazole derivative is prepared by reacting blood at pH 7.0 with an amount of imidazole equivalent to 1-3 times the amount of haemoglobin in the blood. The blood is then heated at 70oC for 60 min and extracted with alcohol/water to yield the pigment, which is obtained in a powder form by freeze-drying. The properties of the haemoglobin-imidazole compound were investigated. It is poorly soluble in water at pH 7 but soluble in 70% alcohol and shows a yellow colour at pH 3-4, orange at pH 5 and red at pH 6-8. Storage in the dark caused little pigment loss, but under illumination at pH 3-5 practically all the pigment was destroyed. However, the compound appeared relatively heat stable as 90% survived a 2h process at 100oC. A subsequent paper by the same group describes modifications to the extraction and preparation procedures and further tests to determine the stability of the pigment. The findings are substantially those reported in the earlier publication.
While searching for alternatives to nitrite in the preparation of fish sausages, imidazole, 5(4)-aminoimidazole-4(5)-carboxamide (AICA) and various amino acid derivatives were used and found to impart colour to the finished products. Imidazole gave a red/pink colour with an orange tint which faded on the surface of the produce unless antioxidant was present. A red colour was formed with AICA and this was attributed to the formation of ferrihaemochromes.
Similarly S-nitrosocysteine was used in comminuted turkey meat as a replacement for nitrite. Its anticlostridial power was less than that of nitrite and it formed more nitrosamines although the colour it formed was comparable with that formed by nitrite.
Carbon monoxide is another ligand which produces a red colouration in haem and imparts a cherry-red colouration to the blood of those poisoned by 'town gas'. Attempts to improve the colour of meat by flushing with carbon monoxide gas have not been successful but it is possible to form a stable haem derivative by the following process. Citrated blood is agitated in the presence of carbon monoxide to saturate the haem with carbon monoxide and then treated at 120oC for 1 h. On cooling, the cooked blood is 'as bright as recently processed blood'. Addition of ascorbic acid to the blood prior to saturation with carbon monoxide gives a final cooked product with a redder hue. Presumably conversion of ferric to ferrous ions in the haemoglobin produces more of the haem(II)-carbon monoxide complex, which is bright red. The process can easily be applied to products containing meat and is claimed to be of use in semi-moist petfoods.
The colouring of spun fibres intended as meat analogues was investigated by Roberts and Lecluse. Inclusion of blood in the protein solution which is spun into fibres coloured the product and, on cooking, gave the fibres the appearance of cooked poultry. When nitrite was included in the protein solution or in the coagulating bath the fibres had typical meat colours and were successfully incorporated into meat products such as ham, luncheon meat and hamburgers. Essentially the same results were reported when solutions containing blood and protein from plant sources were spun into fibres in the presence of nitrite. The fibres resembled cooked meat or ham depending on the concentration of haemoglobin and nitrite.
The methods quoted so far have attempted to stabilise haem pigments by forming stable ligends with iron as the central metal atom. Alternatively the iron atom may be replaced by another metal ion. It is well documented that in porphyrins a series of derivatives containing different metal ions can be prepared, some of which are exceptionally stable. The order of stability of metal derivatives in the tetrapyrrole series has been investigated and the possible use of this method of stabilisation is discussed later.
Two reports from Russian authors cite the preparation and use of a cobalt complex of haematoporphyrin. Reaction of haemin with cobalt acetate in a mixture of acetic and hydrobromic acids gave the water-soluble cobalt complex of haematoporphyrin. Tests in which sausages were coloured with haematoporphyrin (30-50 ppm) and sodium ascorbinate (500 ppm) were successful in that no adverse taste reaction was noted and the colour was described as intense pink. Preliminary animal experiments showed no toxicity.
Included in this section on animal sources is a red colourant derived from a Euphausia crustacean. A Japanese patent describes the extraction of an oil-soluble pigment (presumably a carotenoid) from the crustacean, but no further details are available from the secondary literature source.
Animals appear to be a poor source of colourants. Haemoglobin, and possibly myoglobin, are available but, without some stabilising treatment, their colour degradation on heating or during storage severely limits their use in food except in those products that require a cooked meat colour.
Plant Source
Although there is a multitude of colours in the plant kingdom, their extraction and use in food systems is not an easy task. Logically, an investigation into the suitability of a particular source for producing colourant should start with the isolation of the coloured component. Following identification of the colour, the yield of colourant from the source should be determined. Stability studies should then be carried out to assess the suitability of the colourant for use in foods. From these laboratory investigations it will be obvious whether further studies are necessary. Unless the colourants have some outstanding advantage, e.g. good stability in food or very high tinctorial power, it is generally not worth continuing. Assuming that the source provides a colourant with exceptional properties, further considerations need to be taken into account. A major problem with plant sources is their availability, as most plants are seasonal. Is it economic to set up a process for part of the year and is there sufficient raw material for the process? To overcome this problem of availability (in quantity terms) some by-products have been studied as colourant sources (Table 5). Many of the sources listed under 'Other Sources' would require major planting programmes to provide sufficient material for production purposes. Another consideration is the cost of extraction and processing, which is obviously minimal for an aqueous extraction but will be greater if organic solvents are used and subsequently recovered.
This logical approach to novel sources is rarely presented in literature on the subject. The patent literature is concerned with the framing of an invention rather than detailed consideration of all the potential problems. Conversely, the scientific literature reports straight scientific facts and does not concern itself with financial or legislative aspects. Thus it is left to the individual (or the reviewer!) to pick out which ideas are viable.
General Reviews
Henry divides natural food colourants from plant sources into five types, namely anthocyanins, betalaines, carotenoids, chlorophylls and curcurmin. The sources, pigment structures and some stability problems are then presented. It is suggested that a combination of various techniques (reduction of colour degradation during harvesting and processing; increased pigmentation through breeding; intensification of natural colours) might improve the acceptability of natural colourants in foods. Originally dyes were used for colouring textiles and the origins of these compounds are given. Thus wood and bark from various trees yield a range of yellow, red, blue and black colours which are normally combined with mordants (salts of various metals, e.g. iron, chromium, aluminium and tin) when dyeing cloth to create a fast dye. Fermentation of the leaves of the leguminous plant Indigofera produces indigo, formerly an important article of commerce. Similarly, woad (a blue dye) is produced by fermentation of leaves from Isatis spp. and henna is also derived from a leaf source. Roots and tubers yield the red pigment madder and a yellow pigment morindone as well as the familiar orange-red dye from turmeric, long used to colour food. Of the flower sources, perhaps saffron is the best known. It is derived from crocus stigmas and about 4000 of these are required to produce one ounce of dye. Other sources are seeds

(bixin), resins (gamboge) and lichens (responsible for the characteristic colours of genuine Harris tweed).
Kasumov describe about fifty local Russian plants which are recommended as sources of food colourants. They include fruits such as elderberries and blackcurrants as well as flower petals (rose, poppy) and other sources such as grape pulp and onion skins. In a book on natural food colourants, Kafka review sources of red, yellow and green colourants and give some details on their production and chemical composition as well as a chapter on physicochemical methods for the control of colour in colourants. The use of fermentation, thermal and chemical processes for the production of food colourants from tea leaves and mixtures of tea and red beet is described. It appears that tea polyphenols stabilise the red beet colour by forming complexes and perhaps through inhibition of degradative enzymes. Certainly control of the oxidative enzymes in fresh tea leaves yields dyes with green, yellow or brown colours. Food colours of orange, rose, red and black can also be made. The tea-red beet dyes are not degraded at high temperatures and the colour is pH independent. Polyphenolic compounds may sometimes impart a bitter taste to foods, but it is claimed that the products were organoleptically acceptable and their shelf life was also satisfactory.
Colourants from By-products
Colourant production from by-products has the advantages that there is normally an abundance of the source, it is cheap and in a defined state (e.g. meal, pulp). Further, treatment of the by-product reduces waste and enhances the profitability of a process. Both cocoa beans and cocoa bean shells have been treated to extract red and brown pigment respectively. Cocoa beans contain anthocyanins which, during fermentation and roasting, are oxidised and polymerised to give cocoa its characteristic taste and brown colour. However, extraction of roasted cocoa beans with an aqueous alkaline solution gave rise not to a brown colour but to a red pigment. Extraction of cocoa bean shells with acidified ethanol yielded a material with both flavouring and colouring potential. A variation of this process in which an initial acid treatment at 50oC was followed by neutral or basic extraction gave a brown colour. Because of the intimate relationship between colouring and flavour in cocoa, it would seem that a colourant from this source would also possess the characteristic flavour.
The citrus industry produces large amounts of waste and the extraction of colourants from cold pressed citrus oils has been described. The colourants (presumably carotenoids) are extracted from the oil by solvent and purified. An estimate of the commercial recovery costs was that 1 lb (about 450g) of waste could be treated for 1¢ (1977 prices). No mention is made of any bitter flavours in the extract. Attempts to extract the colour from tamarind seed coats, however, necessitated a preliminary extraction with hot water or hot alkali to remove bitter flavours. Further extraction with alkaline alcohol produced a brown colourant said to be suitable for food use. The amount of this by-product produced per annum was not quoted but it would appear to be a minor source. The same can be said of a patent outlining the extraction of shea nut meal to give food colourants and another patent on the suitability of chestnut, beech or Japanese oak seed cases as colourant sources.
Potential production of a food colourant from a large scale source (sunflower) involves extraction of the seeds with solvents. A few varieties out of the 150 tested contained a red anthocyanin in the black husk. No details of yield are given and since only a few sunflower varieties contain this pigment, it does not seem practicable.
A more widely applicable method of treating vegetable wastes to produce both colourants and flavours is contained in a patent. The basic aim of the process is to decolorise an acid hydrolysate of vegetable matter by passing the solution through a semi-permeable membrane. A light coloured liquid passes the membrane while a dark brown coloured liquid is retained. It is suggested that the retained solution might be used as a food colouring agent.
Gardenia Extracts
There are various references to the use of Gardenia extracts of different colours as food colourants, and for convenience they are grouped together. A stable yellow colourant from Gardenia was stabilised by mixing with sugars (e.g. lactose and dextrin) and spray drying the mixture. This was then refluxed with acetone, filtered and the residue dried to give the stabilised yellow pigment. Similarly, a patent describes stabilisation of Gardenia extract by hydrolysis and reaction with a primary amine to give, in this case, a red substance. The same reaction was reported to proceed under the influence of an enzyme in another patent but no details of the colour formed or its stability are given, although it was claimed that the natural origin of the colour precluded any doubts about the safety of the colourant69. Using soybean legumin protein as the source of the primary amine and a solution of genipin derived from Gardenia, a blue/purple pigment was produced when the solution was boiled for 2 h. A blue pigment can also be manufactured by the aerobic culture of Aspergillus or Rhizopus spp. on Gardenia fruits. When pigment formation has reached a maximum, an ethanol extract of the culture yields a blue pigment. It is also reported that a green colour can be obtained from Gardenia and this is suitable for colouring a canned product. Colour stability was increased by adding vitamin C.
Other Sources
A variety of fruits, vegetables and flowers have been studied as potential sources of colourants. Investigations on the beefsteak plant (Perilla frutescens Crispa) showed that the aerial parts of the plant contained a yellow flavonoid and a red anthocyanin that could be separated by ion exchange chromatography. lshikura also detected anthocyanin in Perilla. The plants may be used for colouring purposes or as food in their own right. It is claimed that the extraction process is economic on a commercial basis.
Following earlier mention of the traditional sources of dyes, it is interesting to note that a patent exists for the use of indigo as a food colourant. Dried indigo plant and two dried herbs were mixed in alkaline solution and heated at 40oC for 1 h. The resulting mixture was centrifuged and the supernatant further treated by adding wheat flour and adjusting the pH to 11 with calcium oxide. Centrifuging produced a supernatant containing the concentrated colouring agent. The effects of the added herbs on the stability of the colour are not clear but it is claimed that addition of herbs makes the colourant more healthy.
Extraction of pigments from coloured strains of vegetables yields a light stable. red pigment from purple corn and red cabbage and a violet pigment from violet sweet potatoes. While these are straight extractions of pigments, a process for producing a brown colour from persimmon polymerises the phenolic compounds (flavonoids, catechin and leuco anthocyanins) to form a polyphenol not found in the whole fruit. The product is water soluble, light and heat resistant and is claimed to be safe, presumably because of its natural origin. In fact the polymerisation is most likely induced by alkaline conditions during the process.
Fruits offer a rich variety of colours and that from cranberries seems to be more stable than other preparations. Below pH 4 a red- colour is observed and the extract has been used to colour jams and beverages with a shelf-life of 6-12 months. Similarly miracle fruit (Synsepalum dulcifum Schum.) yields a red colourant suitable for carbonated (acid) beverages. In the presence of organic acids, especially malic, degradation was rapid. A Russian patent describes the extraction of a red food dye from ripe pokeberries, but gives little indication of the properties of the product. The relatively high amounts of anthocyanin and the lack of alkaloids suggested that the plant Sambueus ebulus L. could be a suitable source of colourants. Besides anthocyanins, polyphenols, sterols and triterpenes were identified but the toxicity of the extract measured by LD50 tests was low at 1200mg/kg.
Flowers are an obvious source of colourants and red dyes from Alcea rosea and hollyhocks have been described. A yellow chalcone isolated from Butea frondosa was shown to consist mainly of isobutrin which was poorly stable in the presence of SO2 and ascorbic acid but showed good pH stability and resistance to heat and light.
Perhaps the most promising colourant from flowers is that derived from 'Heavenly Blue' morning glory. Extraction and analysis of the pigment showed that the anthocyanin peonidin 3-(dicaffeylsophoroside)-5-glucoside was the major component and it retained its colour through the pH range 2-8. The colour changes from purplish/red at acid pH to blue around neutrality but, unlike other anthocyanins, the blue colour is said to be stable at neutral pH. Certainly in the flower itself the pH of the cell sap is around neutrality although subtle pH changes have been noted as the purple/red buds (pH 6.5) open over a 4 h period to give light blue flowers (pH 7.5).
High purity extracts from paprika have also been prepared and suggested as food colourants, but the yellow pigment vulgaxanthine from yellow beets was found to be quite heat labile thus limiting its usefulness in food.
Finally, mention must be made of a source which is not novel-grapes-but the method of production-tissue culture of grape varieties-certainly is novel. The callus induced from the anther of grape plants is transplanted to a liquid culture medium and cultured aerobically. Pigment is then extracted from the culture which can be manipulated to give maximum colour yield during growth by adding various chemicals to the medium or irradiating with light.
Feasibility of Novel Sources
The volume of patent literature concerning novel microbial, animal and plant sources of colourants indicates the potential of the field but, in fact, few of the proposals are workable. Many pigment extracts are no better than the presently available natural pigments and the availability and/or economics of the source material or extraction procedure causes rejection of others.
The author's view is that microbial production of pigments is unlikely in the near future due to the high production and extraction costs and possible legislative problems. Animals offer us the porphyrin nucleus in several guises but unless a friendly stabilising ligand can be found or altered porphyrins containing other metal ions are allowed (see Section 5 on stabilisation) they will only be of use in meat products, most of which are already adequately coloured.
Although plants offer a wide range of pigment colours, few appear to be sufficiently stable to warrant further development work. After all the investigations into different plants, it seems very unlikely that a 'superpigment'with all the required properties will be discovered. Even if it is, the likelihood is that it will exist in some rare species which will require considerable investment for large-scale production. The attraction of manipulating plant cells in liquid culture or plant genes in association with micro-organisms is great until the cost of such methods of production are considered.
Of the many pigments listed in Tables 1, 4 and 5 probably those with the best chance of success are the stable anthocyanins, e.g. that from morning glory, or purpurinidin glycoside and some of the polyphenolic compounds formed under alkaline conditions, the potential of which is discussed in Section 5. Doubtless patents will still be taken out on possible sources of novel colourants but the chances of finding the perfect answer seem as remote as some of the source plants.
STABILITY OF NATURAL COLOURANTS IN FOODS
Having considered novel sources, the next approach to be discussed is the stabilisation of natural colourants in food. Many attempts have been made to retain coloration by adding chemicals or adapting processing conditions (Table 6). For a detailed examination of the many parameters that affect natural colourant stability in food, the reader is referred to the review by Engel which thoroughly covers all aspects of the use of anthocyanins, carotenoids and beet pigments in food systems. The sources of these three pigments together with their chemistry, reaction with food components, applications and limitations are chronicled and discussed. A further series of publications from the Leatherhead Food Research Association examines specific applications such as the use of beetroot red and canthaxanthin in bacon and sausage and the colouring of confectionery products with copper chlorophyll, red beet extract, grape anthocyanin, paprika, annatto, canthaxanthin, b-carotene and apocarotenal, cochineal, riboflavin and turmeric extracts. The above reviews were written specifically for the information of the food industry and are extremely practical in contrast to the vagueness of some patents and the limitations of model systems favoured by scientists trying to isolate the exact cause of instability. Similarly two papers on the stability and use of natural colours in foods describe the utilisation of anthocyanins, b-carotene and riboflavin and red beet powder, copper chlorophyll and cochineal in foods.
A series of papers under the general heading 'Chemistry of natural food colours' contains sections on anthocyanins, chlorophyll, betanin, annatto and cochineal which will be of interest to those using these colourants in foods.
A few specific reports are worthy of mention as they compare the stability of natural colourants against a synthetic colour and report a flavour

side-effect of using natural colourants. Thus the stability of phytolaccanin, betanin and FD & C Red No. 2 (amaranth) was tested in dessert gels, and amaranth was found to be more stable. Again, in a comparison between amaranth and grape pigments, the synthetic colourant was superior and it was found that the natural colourant imparted a detectable flavour to the product although it was quite acceptable in this instance. Factors affecting the stability of natural colourants have been determined in model systems but, as is obvious in the following section, there are conflicting reports. In a complex system such as food, it is difficult to determine which are the primary causes of degradation and which are secondary results of these primary reactions.
EFFECT OF ADDITIVES
Ascorbic Acid and Derivatives
Ascorbic acid has been claimed as a stabiliser for natural colourants and conversely, its presence has been cited as the cause of pigment degradation. With anthocyanins, ascorbic acid sometimes has a protective effect, e.g. when it absorbs available oxygen and thus prevents oxidation of the anthocyanin. In other cases, enzymic attack on ascorbic acid yields hydrogen peroxide which oxidises and decolorises the anthocyanins. Hence addition of ascorbic acid to food products will not necessarily stabilise the colour.
Wrolstad described the effect of ascorbic acid on the colour of an anthocyanin-flavonol model system. It was found that ascorbic acid had a statistically significant effect on anthocyanin loss due to bleaching of the pigment. With Gardenia extract however, ascorbic acid increased the stability of the pigment when used as a colouring agent for canned foods.
Besides ascorbic acid itself, its isomer isoascorbic or erysorbic acid has been used, as have various derivatives of vitamin C. Red beet pigments were stabilised with isoascorbic acid when a concentration of 0. 1% was found to improve colour retention. Similarly, isoascorbic acid was among a number of antioxidants which had a beneficial effect on the keeping-quality of food coloured with phycocyanin. The level used in this case was 0-005 %. A mixture of caramel, ascorbic acid and betalaine was found to produce an enhanced red colour useful for food especially in chewing gum and addition of vitamin C (200-300 mg/litre) to a pink-coloured cider stabilises the colour. Because of the problems sometimes encountered when adding ascorbic acid to anthocyanin pigments, Sweeny devised a method of incorporating ascorbic acid derivatives into a food so that they were available as vitamins but their form prevented degradation of the pigments. Either of the enolic hydroxy groups on ascorbic acid was substituted with a sulphone, phosphate or ethyl group to give a range of derivatives. The esters, such as the 2-0-phosphate, 2-O-benzoate and 2-O-sulphate have been shown to be equivalent to ascorbic acid in terms of vitamin potency. The 2-O-ethylated derivatives are stable to autoxidation and show vitamin C activity albeit at one-fiftieth of the potency of ascorbic acid.
Because of the different chemical nature of colourants and the different physical and chemical environments that foods provide, the general use of ascorbic acid to stabilise natural colourants is not recommended. Only after consideration of each individual case should the use of ascorbic acid be contemplated.
Effect of Metal Ions
Traces of some metal ions, notably copper and iron, have a catalytic effect on the oxidation of ascorbic acid which in turn leads to degradation of anthocyanins. Anthocyanins containing an ortho-dihydroxy grouping chelate metal ions which may alter the colour of the anthocyanin. In canned products anthocyanins of this type may act as anodic depolarisers and react with stannous ions leached from the tin plating by acids. Since the stannous ions are effectively removed from solution by chelation they contribute no charge and acceleration of corrosion is the result. The effect of metal ions on the colour and/or stability of food products has been the subject of several investigations. Coffey studied the stability of the pure anthocyanin cyanidin-3-glucoside and a raspberry juice extract when treated with tin and aluminium ions. Chromatography (HPLC) indicated that a complex was formed with Sn2+ and raspberry juice but not with pure cyanidin-3-glucoside. However colorimetric values suggested that complexes were forming both with Sn2+ /cyanidin-3-glucoside and Al3+ /cyanidin-3-glucoside mixtures. The stability of the products was determined during storage at 24oC and 38oC at pH 2-4. Tin tended to increase colour degradation while aluminium darkened the colour.
A related study on the effect of metal ions on the colour of strawberry puree also showed that anthocyanin degradation occurred in control and tin-containing test samples. However, it was noted that the colour remained in the treated samples despite the breakdown of some anthocyanin and moreover, bisulphite has no bleaching effect on the tintreated samples. Similarly the effect of metal ions in the water supply on the colour of cranberry juice cocktail was studied using colour measurement. The colours produced by metal ions are not always desirable and pink discoloration in canned pears has been attributed to interaction of tin and anthocyanins.
The colouration of anthocyanins by metal ions when a phenolic o-dihydroxy group chelates the ion has been used as a test for the adulteration of wines with elderberry juice. Elderberries contain anthocyanins with chelating powers while grapes contain mainly malvin-a substituted anthocyanin. In glycine buffer at pH 9.23 both sets of pigments are blue, but in borate buffer at the same pH, the elderberry anthocyanins are bright red due to a complex with the borate ion while the grape anthocyanin remains blue.
Effect of Neutral Salts
The dilution of an anthocyanin solution causes loss of colour and a linear relationship between dilution and optical density is not always observed. This has been attributed to seIf- association of anthocyanins. It has been recommended that in the spectrophotometric analysis of anthocyanins, short path length cells should be used if necessary to avoid dilution of anthocyanin solutions. In a grape juice system, inorganic salts were found to increase the colour of the anthocyanin (measured at 520 nm) at pH 3 due to 'an increase in available pigment'. The cause of the increase was not stated. Sodium chlorate produced the greatest colour increase followed by NaNO3, NaBr, NaCl and Na2SO4. The use of neutral salt solutions of high molarity (4 m) to extract anthocyanins has been suggested to prevent losses during isolation.
Effect of Organic Acids
Red anthocyanin pigments from miracle fruit were isolated and tested in carbonated beverages in combination with organic acids. Pigment degradation occurred with all acids and malic acid caused the most rapid degradation. In grape juice organic acids increased the optical density at 520 nm and there was a linear relationship between the number of carbon atoms on the carboxylic acid and the logarithmic value of E520. Maleic acid caused the greatest increase and also increased the colour stability as did valeric acid. Malonic and oxalic acids increased the colour initially but fairly rapid decolorisation occurred on storage.
The known colour variation of anthocyanin pigment with pH has been utilised in a patent for coloured beverages. The pigment is formulated in a powder containing coated organic acid (or coated alkaline salt). On addition of water, the pH gradually changes as the coating dissolves and consequently the colour of the drink changes.
Photoprotection
Light does accelerate the degradation of natural colourants, especially anthocyanins, but it is of secondary importance compared to the losses of anthocyanin due to the effect of heat and/or oxidation. Anthocyanin pigments had doubled resistance to sunlight fading when flavonoid sulphonates were used as copigments. Various sulphonated flavonoids were used and all produced a bathochromic shift of between I and 50 nm. A flavonoid sulphonate (quercitin 5' sulphonate) also prevents discolouration of the pigment rubrolone by sunlight. Stabilisation of the red pigments from Monascus with various phenolic compounds was attempted but only 1,4,6-trihydroxynaphthalene prevented photobleaching of the pigments which were N-glycosyl derivatives of rubropunctamine and monascorubramine.
Miscellaneous Additives
Loss of colour in paprika was prevented by addition of the flavonoid morin or by addition of the products of the browning reaction between different sugars or between sugars and amino acids. In the former case, morin at concentrations under 500 ppm was more effective than rutin or quercetin while in the latter case, inhibition of colour loss occurred when the browning products were incorporated into paprika-coloured foods at levels of 0.005-0-5%. Paprika oleoresin was stabilised by mixing the pigments (dissolved in oil) with an aqueous solution containing 60 % corn syrup solids and 1% polypeptone, vacuum drying and granulating. The granules contained about 12% oil in which the pigment was dissolved and no discolouration was noted during storage at 60oC for 20 days. Laccaic acid was stabilised by admixture with alum and tartrate after which the powdered mixture was used to colour fish cake.
Control of the filling process was claimed to be essential in colouring maraschino cherries with natural colours. The food was adjusted to a sulphur dioxide content of 30 ppm and pH 7 prior to covering with syrup and vacuum exhausting. Colour was then added and the container sealed. Addition of tannins to blackcurrant juice greatly improved the anthocyanin stability when added at levels of 0.4-1.0 g/litre and addition of gelatin to stabilise phycocyanin in foods and beverages has also been reported. Factors affecting the stability of betanin in model food systems were investigated and it was found that decreasing the water activity or adding citric acid or EDTA (10000ppm) increased betanin stability.
Conclusion
While additives can improve the colour stability of natural pigments in specific cases, there are no general guidelines for their use. Thus, ascorbic acid and metal ions can stabilise colour in some situations but their use in unfavourable conditions may actually increase the rate of degradation. Some organic acids may be beneficial, but others enhance instability and the concentrations and/or nature of inorganic salts used to improve the stability of natural colourants are such as to limit their application. Photoprotection using flavonoid sulphonates is possible, but what is the status of the sulphonates? Once again a solution to the problem is dogged by chemical, legislative and economic considerations.
STABLE FORMS OF NATURAL COLOURANTS FOUND IN VIVO
Attempts to stabilise natural colourants in food as described in the previous section appear to be largely unsuccessful. An alternative approach is to study the form in which the colourants exist in nature. Some of the stable forms of natural colourants found in vivo owe their stability to their chemical structure which is per se stable, while others are stabilised by association with other compounds. Mention has already been made of some stable anthocyanins isolated from plant sources. Purpurinidin glycoside extracted from willow is one of these compounds that has the useful property of resisting bleaching by sulphur dioxide whereas most anthocyanins are readily bleached. It has been shown that 4-substituted flavylium salts are resistant to bleaching and purpurinidin glycoside is thought to be a dimer linked through the 4 position. In other words it is the natural form of a compound which has been shown by unnatural means to be stable. The anthocyanin extracted from 'Heavenly Blue' morning glory is also a particularly stable form and is described as peonidin 3-(dicaffeylsophoroside)-5-glucoside. This particular anthocyanin has a very stable quinoidal base which is somehow stabilised by the interaction between the acyl group and the B ring of the anthocyanin. Other blue pigments of this form have also been discovered. The quinoidal base exhibits colour from pH 2-8 although the hue varies from purplish red at acid pH to blue at pH 8.
Other flowers have been studied and it is now realised that the colour of flowers is not due to extremes of pH but to complexes of anthocyanin at the normal cell sap pH of 3.5-5.5. Careful extraction of the blue colour of cornflowers revealed that it was a charged complex which was easily disrupted by acid and contained pectin. Similarly a complex isolated from the syrup of canned blueberries was shown to contain anthocyanin (glycosides of delphinium a and petunidin, 4%) plus a high proportion of polysaccharide resembling pectin (60 %) and a small amount of proteinaceous material (2.8 %). Some metal ions were present, notably aluminium, calcium, iron and potassium, but no tin was detected (ash 2.1 %). The complex had a molecular weight between 77 and 490 Ã- 106 daltons.
Apart from natural sources which contain stable natural colourants, there are also some foods where the colour is known to be stable. The red colour of strawberry jam persists even after several years storage and it has been shown that the pigment composition alters with time. Initially the colour is due to monomeric anthocyanins which, on processing and storage, are converted into polymeric forms which retard pigment degradation and stabilise the colour. Similarly some red wines exhibit remarkably bright colours even after prolonged storage. It has been established that during maturation the monomeric anthocyanins are polymerised maintaining the red colour, giving body and removing the raw taste of young red wines. The polymerised forms also resist bleaching by sulphur dioxide and may therefore be useful as food colourants. Studies on the level of polymers in wine have shown that after one year, 30-40 % of the pigment is present as polymer and after five years, the pigment is totally polymeric. The exact nature of the polymeric material is still unclear but it is probably a mixture of phenolics (including anthocyanins) and nitrogenous compounds such as amino acids.
The stable forms described above are either natural colours or colours which are present in well-known foods that have been consumed for centuries. Under present legislation this would seem to be as acceptable as grape anthocyanins or copper chlorophyll, but their large-scale production presents problems in that the source material is either in short supply or expensive. These examples of stabilised forms of colourants which are derived from accepted sources may be of use when considering the next section.
STABILISED FORMS OF NATURAL COLOURANTS
The mimicking of the native environment in which a natural colour exists is just one way of producing a stabilised form. In this case, the method of stabilisation can be considered 'natural' whereas some of the modifications proposed on the following pages are distinctly synthetic. This section concentrates on the stabilisation of flavonoids and porphyrins. Since these two classes of compounds are widely available, considerable effort has been expended to make them more stable and thus increase their use as food colourants.
FLAVONOIDS
Chemical Features Affecting Stability
This class of natural colourant consisting of flavonoids, anthocyanins and aurones potentially provides the greatest range of colours for food use but the compounds, especially the anthocyanins, tend to be unstable thus limiting their application. Thanks to some fundamental work on the chemistry of the flavonoids, the structural features which confer stability on the molecule are more or less understood. The basic structure of anthocyanin is given in Fig. 3. It is known that hydroxylation of the nucleus at positions 5 and 7 tends to stabilise the molecule whereas hydroxylation at position 3 renders it more labile. Similarly, hydroxy substitution in the B ring leads to instability but this is overcome if the groups are methoxy rather than hydroxy. The substitution of hydroxy groups by sugars or acyl groups also increases stability. Bridle found that substitution of the anthocyanins at position 4 (a position not normally substituted) with methyl or phenyl groups conferred stability on the molecule.
Bearing in mind these known facts of anthocyanin chemistry, what can be done to improve anthocyanin stability taking into account the fact that any process must still maintain the 'naturalness' of the colourant? Firstly, it is possible to specify the desirable features of an anthocyanin for food use e.g. methoxylated B ring, glycosylated or acylated hydroxy groups. It is a fortuitous coincidence that the largest single source of anthocyanin, grape skins (equivalent to 10000 tonnes per year) contain as their major

Fig. 3. General structure of anthocyanin showing numbering system.
pigment malvidin which is one of the more stable anthocyanin derivatives. The acyl and glycosidic substituents of malvidin, besides stabilising the molecule, also give it good water solubility.
With a specification in mind, one could perhaps scan novel sources for suitable derivatives. Considering the large number of novel sources studied there are few which show much promise and those that do are generally available in limited amounts. Is it therefore feasible to consider some synthetic approach to the ideal anthocyanin? Substitution at position 4 would provide stability, but how can this be achieved by natural means, especially as no enzyme seems to operate at this location? Apart from the fact that-an enzyme needs to be found, the cost of isolating the system and then treating anthocyanins with it is likely to be prohibitive. Thus even with a fairly clear idea of the 'ideal' anthocyanin, natural means of attaining it are not easy to achieve. However, the above discussion has only considered the possibilities with monomeric anthocyanins. Some degree of substitution at position 4 can be achieved through dimerisation (as in the naturally occurring purpurinidin glycoside) and the mechanism of condensation, both of which are discussed later.
Self association. In solution anthocyanins are known to self associate and this is thought to stabilise the molecule by physico-chemical means. The exact effect of self association on stability is not known but the nonlinear increase in absorbance that it produces is a useful property (a tenfold increase in concentration increases the absorbance by a factor of 20).
Complex formation. Metal ions have been implicated in the development of flower colour and they particularly affect those anthocyanins with an o-dihydroxy grouping. In nature, anthocyanins probably exist in a complex form which contains metal ions and macromolecules. For instance, stannous ions form a complex with raspberry juice extract although in model systems containing the main raspberry anthocyanin (cyanidin-3-glucoside), no complex was observed thus suggesting that complex formation requires additional components.
Addition of metal ions to stabilise isolated anthocyanins in food products is frequently counterproductive as they also partake in side reactions which can destroy the anthocyanin nucleus. An interesting example of stabilisation appeared originally as a test for wine adulteration with elderberries. In glycine buffer at pH 9.23 both elderberry and grape anthocyanins are blue but, in borate buffer at the same pH, elderberry
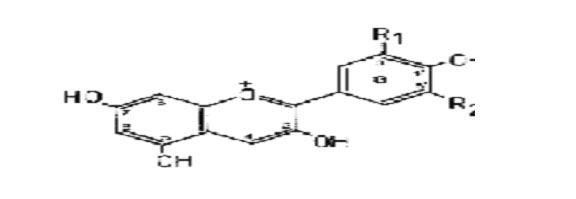
FIG. 4. Postulated structure of the borate complex formed with elderberry anthocyanins.
anthocyanins remain red due to complex formation with borate (Fig. 4). Thus wine can be readily tested for the presence of elderberry juice. Preliminary experiments in this laboratory confirmed the original findings, but attempts to form a stable complex with ions more suited to food use than borate were unsuccessful.
The formation of an inclusion complex of anthocyanins with cyclodextrin has also been reported. The anthocyanins callistephin and chrysanthemin were added to a 1% solution of b-cyclodextrin at pH 2. After 60 min the absorbance at 498 nm was 51.2% of the original value and at 512 nm 64 %. a-Cyclodextrin had no effect on chrysanthemin and only a slight effect on callistephin. The decreased absorbance was reversed when the solutions were acidified to pH [ 0.05 showing that the loss of colour was reversible and suggesting that an inclusion complex had formed. The authors further suggested that the inclusion complex protected the anthocyanin from degradation. Considering that anthocyanins probably exist naturally as complexes, it is surprising that so little published work is available on this potential method of stabilisation.[/P]
Copigmentation. This term describes another state in which anthocyanins exist in nature i.e. intimately mixed with other phenolic compounds. In fact combination of anthocyanins and flavonoids in the correct ratio and at the correct pH produces the spectra observed in flowers and this explains the multitude of colours which exist at the pH of cell sap. This phenomenon not only affects the colour of the anthocyanin but also helps stabilise it against light, which is not surprising considering the stability of flower colours to sunlight. For this reason, processes for stabilising anthocyanins with a variety of flavonoids have been patented. Solutions of apigenin, primetin, tricin, rutin or myrecitin were prepared with water, propylene glycol or glycerine as the solvent. Anthocyanin was then added and the copigmented product was reported to be of stable composition, resistant to light degradation and to possess good brightness and colour. A similar patent application described copigmentation of anthocyanins by mixing them with gallic, digallic or tannic acids dissolved in methanol, ethanol, ethylene or propylene glycol and acetone at 10-60oC and pH 2-5. The copigments were found to be light resistant.
Timberlake reported that the colour of anthocyanins was augmented when mixed in solution with acetaldehyde and catechin. Of the many aldehydes and ketones tested with mixtures of catechin and anthocyanin at pH 3.5, acetaldehyde was the most effective and the different properties of the product were attributed to condensation between catechin and anthocyanin with the acetaldehyde acting as the bridge between the two. Condensation reactions are further discussed in the next section.
Work by Hrazdina on the copigmentation of anthocyanins showed that the colour intensity and the stability of the anthocyanin-rutin complex were greater than with anthocyanin alone. The pH at which complexing takes place depends on the nature of the anthocyanin. For 3-acylglucosides, pH 4-4.5 was suitable whereas pH 3.1 was preferable for 3,5-diglucosides. Early work by Harborne explained the nature of the blue colour of irises which is due to copigmentation between mangiferin and anthocyanins. Copigmentation generally produces blue or mauve colours.
Condensation. Whereas copigmentation is generally thought to be the association of molecules without the formation of covalent bonds, condensation implies that covalent bonds are formed with the liberation of water. Condensation reactions in anthocyanins take place naturally during the production of jam or the maturation of wine when monomeric constituents undergo polymerisation and produce pigments which are more stable than monomeric forms (especially to sulphur dioxide discoloration). The mechanism of condensation of synthetic flavylium salts has been studied by Jurd and the same author has characterised the products obtained by the condensation of flavylium salts with phloroglucinols, catechin or themselves (see Fig. 5). The structures of the products are of interest because it has already been shown that modifications at certain loci on the anthocyanin nucleus benefit stability. The condensation of flavylium salts with phloroglucinol gave colourless products due to the position of the covalent linkages. Phloroglucinol links to C2 and C4 of the anthocyanin and it is the linkage at C2 which leads to loss of colour in the same way that binding of -OH (carbinol form) or sulphur dioxide at C2 causes decolorisation. This type of linkage is of no

FIG 5. Proposed structure for condensation products of flavylium salts with phoroglucinol (above) and self condensation (below).
use when the aim is to produce stable anthocyanins possessing colour characteristics. Condensation of a synthetic flavylium salt with catechin however, produced a red/orange colour, albeit as a precipitate, and this is due to a different kind of linkage, namely between C4 of the flavylium salt and C8 or C6 of the catechin. In this case C2 remains unsubstituted. Dimerisation of flavylium salts is also effected through C4 to C8 or C4 to C6 linkages. While these experiments with synthetic compounds are important in the understanding of condensation reactions, they will not produce natural colourants. Synthetic derivatives are a convenient tool for the chemist but are unlikely to be accepted as natural colourants' for food use. Several reports on the production of food colourants by means of condensation reactions have appeared. Most of them have not used anthocyanins but have used other polyphenolic compounds and the reaction that occurs is essentially that of enzymic browning. Catechin can be dimerised by atmospheric oxygen either under alkaline conditions or by the enzyme polyphenoloxidase. Further exposure to oxygen and heating causes the dimers to polymerise. Catechin is colourless but the dimer exhibits a pleasant orange/red colour which turns browner as polymerisation continues. The colour will survive a retort cycle at normal food pH and it is only sensitive to extremes of pH. Another property of the dimer is that it readily adsorbs to macromolecules and, in its preparation, the enzyme polyphenoloxidase is in fact partially inactivated due to this binding. In a food product containing several varieties of macromolecules, the dimer will attach itself to the product and colour the food rather than the surrounding liquid.
Two books describe how fermentative and thermal/chemical processes can produce novel food colours from tea and red beet. Manipulation of the tea fermentation yields yellow, green or brown dyes and mixing the tea polyphenols with red beet extracts gives a red dye. The polyphenols stabilise. the beet pigments and they are not degraded at high temperatures: moreover their shelf life is reported to be good. There appear to be no flavour negatives despite the astringent nature of tea polyphenols and the authors suggest that the inclusion of substances that have vitamin P activity improves the nutritional quality of the food.
The same basic idea is also described in two Japanese patents which give some novel sources of phenolic substances that may be polymerised to give dyes. Thus a brown polyphenolic colouring agent which is water soluble, heat resistant and safe was prepared by reacting the phenolic components of persimmon in neutral or alkaline conditions. Alternatively, chestnut, beech or Japanese oak seed cases yield a polyphenolic material that gives a brown or red/brown colour when heated in neutral or alkaline solutions.
This method of producing stable colourants has many attractions. Firstly it is a totally natural, accepted reaction responsible for enzymic browning in fruits and tea production. The products therefore, have been widely used over a long period of time without any obvious signs of toxicity. Production of the colourant is facile requiring atomospheric oxygen and either alkaline conditions or an enzyme, polyphenoloxidase, which is widely distributed in nature. As in tea fermentation, it may not be necessary to isolate the phenolic compounds and the enzyme before reaction. Rather, simple mixing of crude sources may produce the desired effect. It seems likely that precise control of the reaction conditions could yield various colourants and their binding power to macromolecules could be used to advantage during synthesis to prevent inactivation of the enzyme. A further possibility would be to use mixtures of anthocyanins and polyphenols to produce novel colours by natural means.
Chemical modifications. Although chemical modification is probably unacceptable in the preparation of natural colours, there are some patented processes which improve the stability in food. The reduction of naturally occurring flavanones (after acetylation) gives flavans which may be of use as food colours. Reduction of flavanones with sodium borohydride followed by oxidation with benzoquinone derivatives gives 3-deoxyanthocyanindins. Again use as colourants has been suggested.
Summary. The present use of flavonoids as food colourants is limited to anthocyanin extracts from grape processing. These preparations are largely unsuitable for food use by themselves. However, the preceeding sections have outlined some methods which might improve their appeal. From the wide range of ideas listed, the following have been selected as they appear feasible methods of stabilisation within the constraints of retaining natural status.
- Complex formation. Anthocyanins occur naturally as complexes which are relatively stable. Studies on pectin/anthocyanin complexes may be useful.
- Copigmentation. In formulating a product containing anthocyanin colourants, the inclusion of a copigment would augment and stabilise the colour.
- Condensation. This seems the most likely way of producing acceptable, natural colourants for the reasons stated previously. Considerable research to study the production of the polymeric colours and their stability in food products needs to be carried out.
Porphyrins
Chlorophyll is the porphyrin most widely used as a natural colourant. Its widespread occurrence in photosynthetic tissue and its lability have prompted many investigations concerning stabilisation of the molecule. Breakdown of chlorophyll occurs in one of three ways which are listed below in order of importance:
- Loss of magnesium.
- Loss of phytol.
- Oxidation and allomerisation.
Loss of magnesium follows denaturation of the protecting protein in the chloroplast. Thereafter, the rate of magnesium loss is proportional to the square root of the hydrogen ion concentration. Several processing techniques to minimise chlorophyll loss involve alkaline blanching and soaking media and the best known is probably the Blair process for peas. Loss of magnesium yields pheophytin which is an olive-brown colour. Loss of phytol causes no colour change but the resulting chlorophyllide is then less stable and is also water soluble which means it is easily lost to aqueous media. Oxidation and allomerisation are changes that are most important during storage and lead to colour loss.
Attempts to stabilise chlorophyll have centred around the substitution of the normal magnesium ion, following the discovery that the 'regreening phenomenon' observed in some products is due to zinc and copper ions in the food being incorporated into the chlorophyll nucleus. Thus, it is possible to substitute magnesium in the original molecule for zinc or copper by control of the conditions of thermal processing. The zinc and copper derivatives maintain the basic green colour of chlorophyll but there is a slight bluish tinge which is particularly noticeable in concentrated solutions. Substitution of magnesium with copper is also practised in the preparation of the copper chlorophylls in water-soluble. (without phytol) and oil-soluble forms. Logically, however, it is possible to substitute many other metal ions for magnesium. In a study on chlorophyll, Lamort devoted a chapter to metal exchange in chlorophylls and claimed from spectrophotometric data to have synthesised the platinum, palladium and cobalt derivatives of chlorophyll. There are no data on the stability of these chlorophylls but experiments on the closely related tetrapyrrole systems have shown that many ions can be substituted and, of the ones tested, the order of stability was as follows:
Pt ] Pd ] Ni ] Co ] Ag(II) ] Cu ] Zn ] Mg
] Cd ] Sn ] Li ] Na ] Ba ] K ] Ag(l)
Hence, if the order of stability in chlorophylls is the same as in the tetrapyrrole series, cobalt and nickel derivatives of chlorophyll will be even more stable than the copper derivative (platinum, silver and palladium derivatives can be disregarded because of their prohibitive costs). Whether these cobalt and nickel derivatives would be accepted as natural and whether their synthesis is feasible are questions that remain to be answered. One of the common metals missing from the above order of stability, iron, has been incorporated into phytochlorin171. A solution of phytochlorin in acetone was refluxed in the presence of reductants and iron salts for three hours and gave iron chlorophyll in good yield (2 g phytochlorin gave 1.9 chlorophyll). The stability of this derivative in comparison to the copper, zinc or magnesium versions is unfortunately not documented. From the legislative point of view, iron chlorophyll would seem to have a greater chance of acceptance over derivatives such as cobalt or nickel because of the already high consumption of iron porphyrin (haem) compounds in food.
The metal ion in isolated chlorophyll is unstable because, although it is satisfactorily held by the four nitrogens of the tetrapyroole ring in one plane, it lacks ligands in the other planes. Thus it is susceptible to attack. Presumably, the protecting protein in nature wraps around the chlorophyll molecule and stabilises it by providing additional ligands in the correct planes. One consequence of this lack of ligands is that chlorophyll tends to aggregate in solutions and form stacks of molecules. This is an annoyance to scientists studying the molecule and in an attempt to prevent aggregation, the phytol chain of chlorophyll was replaced with a chain containing a heterocyclic ring. This has sufficient length and flexibility to bend over and form a fifth intramolecular ligand to the magnesium ion which prevents aggregation and stabilises the molecules. While this particular method is unsuitable for food use, the principle of stabilising chlorophylls by providing stabilising ligands has received little attention.
Besides chlorophyll, there are other porphyrins that may have use as colourants. Haem is the most common but its lack of heat stability has limited it use. There are however many other porphyrin derivatives that have not beep studied as food colourants although a great deal is known about their chemistry. Unlike the chlorophylls where the replacement of the metal ion changes just the hue of the green colour, porphyrins exhibit wider colour variations with a change of metal ion. Methods of preparing metalloporphyrins are available and it is claimed that 100% conversion can be achieved in five minutes. Porphyrin derivatives of zinc, copper, nickel, cobalt, iron, chromium, manganese, vanadium, mercury, cadmium, lead, tin, magnesium, barium, calcium, palladium and silver have been prepared. Little is known about the colour stability of these compounds because they were not synthesised with food colourants in mind.
As ever, the question 'are these acceptable in food?" must be asked. Certainly derivatives containing toxic metals can be discounted, but there are a number of metaIloporphyrin complexes that occur naturally and their existence poses the question 'how are they biosynthesised?'. Lowe and Phillips observed that the rate of incorporation of copper into protoporphyrin was increased by a factor of 25000 when sodium dodecyl sulphate (SDS) was added and by a factor of 500 000 with SDS + 8-hydroxyquinoline. This suggested that a micellar system was involved and in the same year the enzyme that catalyses the incorporation of iron into protoporphyrin was isolated from rat liver and its metal specificity studied. Iron and cobalt were incorporated into protoporphyrin by the enzyme but other metals caused inhibition. An enzyme from erythrocytes was shown to catalyse cobalt (II) and zinc (II) incorporation into porphyrin at 2% and 10% of the rate observed with iron (II). However, the same authors reported that the liver enzyme incorporated both cobalt (II) and iron (II) at the same rate. Thus it has been shown that cobalt derivatives of porphyrins are formed naturally by enzymes, so conceivably these compounds could be classed as natural. Obviously much more work on the stability of cobalt porphyrins versus iron porphyrins needs to be done to assess the amount of any advantage. It is worth noting that human ingestion of cobalt up to a dose of 150 mg per day is considered healthy while 500mg per day is a toxic dose.
At this point it seems appropriate to mention the production of coproporphyrin derivatives for use as food colours. A Japanese patent describes the products of a reaction between the alkyl ester of coproporphyrin III and alkali metal hydroxides in various solvents. A major advantage of the process is the high degree of water solubility of the products. No data are available on their stability.
Another patent describing treatment of phycocyanin for food use is also of interest. Phycocyanin when extracted from Cyanophyta consists of a protein plus the chromophore (phycocyanobilin) linked by a peptide group. In many applications, the protein becomes denatured and precipitates which also causes precipitation of the colour. Treatment with protease prior to use degrades the protein and prevents precipitation. Chlorophyll also occurs as a protein complex where the protein is thought to stabilise the molecule by providing additional ligands. Heating causes protein denaturation and chlorophyll is then more susceptible to attack. Whether limited proteolysis of the chlorophyll/protein complex would prevent loss of the ligands due to denaturation is an idea worthy of consideration.
Others
This section deals with natural colours other than porphyrins or flavonoids and with both chemical and physical methods of stabilisation.
Carriers are frequently used with natural colourants to aid solubility and to standardise the tinctorial power of the preparation. Dextrins are popular because of their easy solubility, but other carriers may confer benefits besides solubility on the colourant. Thus a carmine dye was prepared by adsorbing carsamine onto a carrier of finely-divided cellulose containing 2% chitosan. The preparation was dispersible in water and gave a clear carmine colour without bleeding.
Carriers have also been used to stabilise light sensitive colourants. Carbonates, silicates, cellulose, starch, alginate, polyamides or polyacrylates of particle size
1-100mm were used and the colour properties of the adsorbed pigments were maintained. The use of an alum and tartrate carrier has been claimed to stabilise the colour of laccaic acid. A mixture of the dry components was powdered, dissolved in water and then used at the 10% level to colour fish cake. The advantages of the method and the relatively high dosage are not explained. Similarly, the use of a sodium tartrate/ alum/sodium bicarbonate carrier has been suggested as a means of producing stable forms of anthraquinone and carotenoid pigments for food use.
Carriers have also been used to produce emulsions of natural colours. A mixture of natural colour, oils and hydrophobic colloidal material (e.g. gum arabic or pectin) was emulsified and then spray dried yielding a powder that was used in colouring sucrose-containing powdered food materials. Although no mention of encapsulation was made in this paper, it seems likely that the process of spray drying an emulsion will tend to encapsulate the colour and protect it. A method for stabilising curcurmin by encapsulation has been patented. Curcurmin is destroyed at alkaline pH and thus the aim of encapsulation is to maintain the pH between 3-5 and 4.5. Mixtures of curcurmin, organic acid, buffer and a suitable encapsulating agent are spray dried to trap the colourant in an environment which favours stability. Since the stability of natural colours in living tissue is due to their immediate environment, this approach to stabilisation is both sensible and feasible because it retains the naturalness' of the product.
Besides carriers, other compounds can also assist in stabilisation. Phycocyanin, which as noted previously, tends to precipitate out in food systems, can be stabilised by adding gelatin to the formulation and adjusting the pH to 3.5 with citric acid. This treatment prevents precipitation. Another approach is to use iron complexes of hydroxy-4-pyrone structures to give novel red colours which are claimed to be suitable for food use. Two recent patents on the stabilisation of beet pigments have appeared. One used an ascorbic acid derivative in conjunction with sodium hexametaphosphate while the other used certain processing conditions to yield a stabilised pigment.